Introduction
Micro and nanosystems synthesized from polymer materials are gaining attention in biomedical applications such as the design of biosensors, construction of imaging systems, synthesis of drug-carrying, and drug targeting devices. 1,2,3,4,5)
Nanogel-particles of polymer gels having dimensions in the order of nanometres are encouraging novel pharmaceutical carriers for biologically active compounds and biomacromolecules.6,7 The advantages of these systems include simplicity formulation with the drugs, stability of the resulting formulation in dispersion, and high loading capacity that depends on the functional group present in the polymeric unit. The most beneficial feature of nanogels is their rapid swelling/de-swelling characteristics. 2,8) Nanogels, which are 20 to 200 nm in diameter, are effective in avoiding the rapid renal exclusion but are small enough to avoid the uptake by the reticuloendothelial system.2 Furthermore, nanogels can be chemically modified to incorporate different ligands for targeted drug delivery. The in vivo and in vitro studies suggest that nanogels can be used for efficient delivery of biopharmaceuticals in the cell as well as to increase drug delivery across cellular barriers.9
Several techniques have been described for the synthesis of polymeric micro and nanogels.1,10 Most of them can be classified into two groups. The first one is based on concomitant polymerization and crosslinking (where the substrates are monomers or their mixtures). The second group involves methods based on intramolecular crosslinking of macromolecules (where the starting material is a polymer rather than a monomer). However, the use of radiation was proven to be suitable for producing hydrogels (macro, micro, nano) for biomedical purposes. The use of ionizing radiation to obtain nanogels has important advantages in comparison with conventional methods. These include easier production process, synthesis and sterilization steps at the same time, elimination of harmful compounds which are difficult to remove, eco-friendly and might as well reduce manufacturing expenses. 11,12,13,14) Therefore, the polymer nanogels prepared using a gamma facility boast excellent properties such as high purity and, as a result the main area of its applications include wound dressing, drug delivery systems, implants, “smart systems” amongst others.10,11,14
Particularly, in this paper, the poly (vinylpyrrolidone) (PVP) nanogels can be obtained by gamma radiation based on the intramolecular crosslinking of polymer chains in aqueous solutions. This research is focused on the study of the influence of total absorbed dose on both formation process and nanogel´s properties. Simultaneously, we evaluated the biocompatibility and immune response of these nanogels considering their potential use as biomaterial. The nanogel characterization was carried out by Scanning Electron Microscopy (SEM), Attenuated Total Reflection-Fourier Transform IR Spectroscopy (FTIR-ATR), Dynamic Light Scattering (DLS), and Viscosimetry techniques. In order to evaluate the biological characteristics of nanogels, the yellow 3-(4,5-dimethythiazol2-yl)-2,5-diphenyl tetrazolium bromide (MTT) and Nitro blue tetrazolium (NBT) assays were performed.
Experimental section
2.1. Chemicals
Polyvinylpyrrolidone-PVP (K-90 >99.99%,120 kDa, Sigma-Aldrich) solutions were prepared with deionized water and in order to remove any dust particles and/or polymer aggregates, solutions were filtered subsequently through filters of 0.45 and 0.2 µm pore size. The pH and conductivity of the solutions were found to be 8.02 ± 0.05 and µ= 2.4 µS/cm 0.5% of the measured value. The chemicals used in this study were mostly reagent with high analytical grade and used as received (Sigma-Aldrich) without further treatment.
For in-vitro cell culture, the Vero cell line (ATCC CCL81) was derived from the kidney of a normal adult African green monkey. It was supplemented with a growth medium 199 (5% fetal bovine serum).
2.2. Synthesis of nanogels
The polyvinylpyrrolidone nanogels were obtained by the described method in the literature. 15) The aqueous PVP solutions (0.05 - 0.3% p/v) were subjected to irradiation dose ranging from 3 - 22 kGy, previously these solutions were saturated under Argon flow. Gamma irradiation was carried out with a panorama 60Co source (ISO GAMMA LL-CO) at room temperature. The irradiation process was monitored by Fricke dosimetry and film dosimeters using Perpex.
2.3. Characterization
Measurement of viscosity
According to the method described in ISO 1628-1: 1998 (E) and NC 30-13 norms, 16,17) the viscosimetric study was carried out in an Ubbelohde viscometer. From the obtained flow-time measurements, the reduced viscosity of unirradiated and irradiated PVP samples was calculated, particularly the intrinsic viscosity was calculated using Huggins equation (1) and total absorbed dose influence was evaluated on it.
Chemical structure by FTIR-ATR
By FTIR-ATR the chemical structure of unirradiated and irradiated PVP samples was studied. Sample drops were deposited onto the diamond crystal and the analysis was performed. The study was developed with a Perkin Elmer Spectrum One spectrometer in reflectance mode, with 20 scans and a resolution of 2 cm-1 in the range of 250 - 4000 cm-1, at room temperature.
Morphology by Scanning Electron Microscopy (SEM)
The morphology of produced particles was investigated using a Scanning Electron Microscope (JEOL JSM-6390LV), operated with an accelerating voltage of 0.5 - 30 kV employing a tungsten filament. The amplification was from 5X until 300.00X. 0.2 mg of lyophilized nanogel was put onto SEM support and coated with a thin layer of gold to have a conductive material for SEM analysis.
Measurement of Dynamic Light Scattering (DLS)
The molecular weight M w and hydrodynamic radius R h of unirradiated and irradiated samples of PVP were performed using a particle analyzer (DelsaTMNano).
The Mw was estimated according to equation (2) where D is the coefficient of diffusion of the sample and 𝛼, 𝛽 are constants that represent the structural and solvent effects in the translational movement of the particles respectively.
Also, the concentration of intermolecular crosslinks [intermolecular crosslinks] was calculated according to equation (3) where M w0 , and M w denote the molecular weights before and after irradiation and C polymer is the polymer concentration. (18)
2.4. Biological assays
Cytotoxicity evaluation: MTT test
The micro and nanogels for biomedical purposes have to be manufactured from biocompatible materials or to achieve the lower harmfulness into the organism. The biocompatibility of PVP nanogels was evaluated on the Vero cell line by MTT test.19 The cells were seeded onto 96-well plates in a confluent monolayer way. Subsequently, the cell culture medium was removed from 96-wellplate and was added 100 µL of each nanogels dilution for a total of five replicates by dilution. Previously, the nanogels were freeze-dried and sterilized by gamma radiation and finally were dissolved in maintenance medium (2% fetal bovine). The cells were incubated for 48 hours at 37 0C and 5% CO2. To assess the cytotoxicity, 10 µL MTT (5 mg/mL in PBS) were added to the plate and incubated in the dark for 4 hours at the above conditions. The control Vero cells were kept in maintenance medium (2% fetal bovine). In the end, the cells were solubilized with 100 µL dimethyl sulfoxide and the solubilized formazan was measured by UV at a wavelength of 520 nm using a reference at 620 nm.
Neutrophils separation
Isolated neutrophils were obtained from venous blood of healthy donors, who signed the informed consent approved by the ethical committee. Cell isolation was performed by differential centrifugation in Percoll density gradients.20 In brief, 12mL of blood was placed on the density gradient of 12mL of 60% Percoll above 12 mL of 70% Percoll and centrifuged at 1300 rcf for 15 minutes. Subsequently, the layer rich in neutrophils was collected in another tube and washed twice with HBSS followed by centrifugation. The residual erythrocytes were eliminated by hypotonic lysis buffer and centrifugation. The neutrophil pellet was evaluated by cell counting, viability, and purity analysis. Only samples presenting >107 cells, > 98% viable, > 97% neutrophils were considered acceptable for the next steps.
Innate immune response evaluation: neutrophil activation NBT test
Following the isolation, neutrophils were separated into four comparable aliquots so that each condition had 6600 cells/μL and suspended in HBSS with calcium and magnesium and plasma obtained from the same donor. One of these aliquots was incubated only in HBSS and plasma as a negative control, one with 100 nmol/L fMLP (bacterial peptide to activate neutrophils,21 used as positive control) the third aliquot was incubated with 200 μg/mL TiO2 nanoparticles, and the fourth one with 100 μg/mL of the nanogel described in this work. All the samples were incubated in thermomixer at 37 °C and 300 rpm. After 10 minutes an aliquot of 2.38x105 cells in 36 μL of each condition was transferred to another tube and incubated with Nitroblue Tetrazolium (NBT) in thermomixer at 37 °C, 300 rpm for 20 minutes. After that, an aliquot of 6 μL was taken from each sample and put on glass slides, fixed with methanol, counterstained with safranin and washed for optical microscopy analysis. Neutrophils were evaluated for activation patterns (intensity and distribution of formazan crystals) and activated cells were counted in a total of 300 cells per replicate.
Results
3.1 Viscosimetric Analysis
The synthesis procedure of nanogel formation was monitored by viscosimetric measures. Using flow time measurement of irradiated and unirradiated PVP solutions, the reduced viscosity was calculated (Table 1).
The reduced viscosity of unirradiated solutions considerably decreases after irradiation in all intervals of studied dose and concentrations (Table1). There was a downtrend of these values until 15kGy, after that it began raising.
Subsequently, to be more accurate, the intrinsic viscosity for irradiated and unirradiated PVP solutions was calculated using the plot of reduced viscosity and each concentration value through the Huggins equation (Fig. 1). The intrinsic viscosity value was 108.79 ± 1.99 L g−1 for the unirradiated solution.
A similar behaviour was observed in the values of intrinsic viscosity of irradiated solutions which significantly decreased in comparison with the unirradiated ones. The lowest value was observed at 15kGy. The viscosity results evidence a strong dependence of total absorbed dose.
3.2. Analysis of Dynamic Light Scattering (DLS)
DLS analysis data shows the changes of the cross-linked macromolecules dimension. Also, in order to know the mainly crosslinking way in nanogel formation. The concentration of intermolecular crosslinks [intermolecular crosslinks] was estimated using equation 3. The results for the PVP solution (0.1%) are shown (Table 2).
Table 2 Values of the R h , M w and calculated [intermolecular crosslinks] depending of total absorbed dose for PVP solution
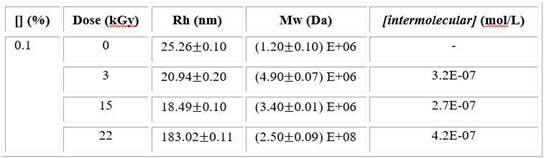
At low dose, the Rh slightly decreases and Mw strongly increases compared to unirradiated sample. When dose is around to 15 kGy, the Rh shows the lowest value and the Mw increases lightly while both magnitudes rapidly raise at higher dose. This trend was also observed in viscosity results. The lowest value of intermolecular concentration was found at 15kGy.
3.3. Analysis of chemical structure
To study of synthesis mechanism or changes in chemical structure that take place as a function of the irradiation dose used FTIR-ATR technique was performed. The ATR spectra of irradiated and unirradiated PVP solutions were measured. The FTIR-ATR spectra of unirradiated solutions did not evidence considerable changes compared to the spectra of irradiated solutions (Fig. 2). Besides, there are no substantial changes of FTIR-ATR spectra influenced by irradiation dose at the same concentration value.
3.4. Analysis of morphology
Figure 3 shows SEM images of 0.1% PVP samples at different dose. Image A shows a predominantly intermolecular crosslinking, low porosity and high agglomeration, a similar result was obtained in the case of image C, where it was observed the morphology of reticulated polymer with low porosity and lightly ruggedness comparable to a macrogel instead of the nanogel structure. In image B, obtained nanogels are shown with an elliptical or spherical shape with different particle sizes. These results corroborate the obtained results by DLS. It is important to say that the agglomeration effect of nanogels in solid-state influences on the evaluation of particles size by SEM and as a result, it is not totally reliable, we suggest the use of Transmission Electron Microscopy (TEM) to achieve a larger accuracy result,22 in accordance to DLS results.
3.5. Analysis of biocompatibility
To know the suitable nanogel concentrations for pharmaceutical or biomedical applications we evaluated the cytotoxicity of nanogels in the Vero cell line. The nanogels cytotoxicity was studied at a concentration range from 0.0625 to 1 mg/mL. Cell viability was evaluated through the colorimetric MTT assay, where the formazan production from the reduction of MTT occurs in metabolically active cells. Median values and standard deviation were calculated for each treatment. Normality, variance homogeneity and one-way analysis of variance (ANOVA) tests were performed. Median values of each treatment were compared with the control using Dunnett test (p < 0.05). The results were analyzed using the computer program GraphPad Prism (Graph-Pad v7 Software, Inc, USA).
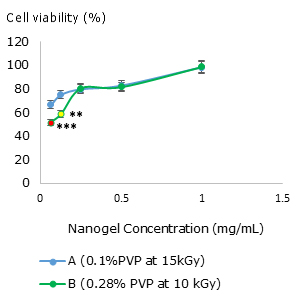
Fig. 4 Influence of nanogel concentration on Vero cell viability for (A) nanogel synthesized at 0.1% PVP at 15 kGy and (B) nanogel synthesized at 0.28 % PVP at 10 kGy. The asterisks mean a significant difference compared to the control ** (p= 0.01) and ***(p= 0.001).
As shown in figure 4, both nanogels, did not represent substantial toxic effects on the Vero cell line in concentrations between 0.25 and 1 mg/mL. Nanogels synthesized at 10kGy and 0.28% of PVP (B) at low concentrations of 0.0625 and 0.125 mg/mL presented significantly lower viability than the control, although cell viability is higher than 50%. The nanogels synthesized at 15 kGy and 0.1% of PVP (A) did not show any cytotoxicity effect on Vero cells. Nevertheless, this cell line diminishes the cell viability to 66.67% (A) when it is applied in low concentrations. In general, increasing the nanogel concentration raises the living cell percentage.
3.6. Analysis of the innate immune response: neutrophils respiratory burst activation
The reduction of NBT by reactive oxygen species (ROS) produces formazan crystals. 23) These are accumulated inside the cell as well as deposited near the cell surface when these cells produce high concentrations of ROS in the presence of pathogen-related molecules, such as fMLP, and foreign particles (TiO2). Therefore, they were used as a marker of neutrophil activation.
The microscopy assays results (Fig. 5) showed that fMLP caused an intense neutrophil activation to 68.0% of the cells contained extensive formazan crystals as shown (Fig. 5 B), only 33.0% of neutrophils exposed to TiO2 (Fig. 5 D) were activated. Only baseline production ROS was detected in the negative control samples that showed 17.2% of cells with low amounts of formazan (Fig. 5 A). Finally, neutrophils incubated with nanogel showed several activated cells similar to the TiO2 condition (38.0% of the cells contained formazan), but a lower intensity activation pattern than in the TiO2 case was observed, suggesting a softer activation by nanogels (Fig. 5 C).
Detection of superoxide anion production using NBT assay. (A) negative control cells confirm the quiescent state; (B) cells exposed to the pro-inflammatory peptide fMLP (chemical positive control); (C) neutrophils exposed to nanogel particles and (D) neutrophils incubated with TiO2 particles.
Discussion
The role of nanogels in biomedical and pharmaceutical sciences is ahead consideration in the last few years for their wide applications range.2,8,24 Several researchers have studied the synthesis, characterization, functionalization, and potential as nanomedicine carriers of nanogel.22,25,26,27,28
In this research, the synthesis procedure of nanogel formation was monitoring by viscosimetric measures. The viscosity data are related to size changes of polymer coil and, as a result to inter- or intramolecular crosslinking during the macro/micro/nano-gel formation.29,30 High values of dose and diluted concentrations contribute to intramolecular crosslinking and, the possible formation of nanogels occurs. It is important to stress that this process could be accompanied by a slight increase in molecular weigh. 29 The results show a predominantly intermolecular cross-linking at dose lower than 10 kGy and from 15 to 22 kGy whilst at dose close to interval of 10 -15 kGy (Fig. 1) a coil shrinkage resulting from mostly intramolecular crosslinking occurs. The last effect indicates possible formation of nanogels, according to the literature.30 Although the viscosimetric studies suggest the nanogel formation approximately at dose ranges from 10-15 kGy, it is necessary to corroborate these preliminary results using a more accurate assay. 29,30,31)
By DLS the hydrodynamic behaviour of macromolecules is studied reliably. The changes of Rh involve variations of dimension of internally cross-linked macromolecule. This phenomenon describes the macromolecule dimension more direct than viscosity data.30
At low dose, the Rh slightly decreases suggesting changes in polymer size but due to the fact that Mw increases considerably a stronger contribution of intermolecular reactions are present and it favours a macrogel structure. It is possible to corroborate this statement analysing SEM images (Fig. 3 A). However, the Rh decreases prominently and the Mw increases lightly at 15 kGy indicating lower intermolecular recombination (Table 2). Then, single macromolecules mainly intramolecularly crosslink and nanogels are formed, in accordance other researches.18,29 Since the lower [intermolecular crosslinks] value corresponds to 15 kGy, this result validates the above statement. The long irradiation times favour encounter of coils and, as a result micro/macrogel are formed, even below overlap concentration (C*). This fact take place mainly at highest dose increasing the Mw (Table 2).14,32 By SEM analysis (Fig. 3) is possible to verify all DLS results in agreement to other studies.18,33 Consequently, the total absorbed dose has a considerable influence on crosslinking.
On the other hand, the chemical structure of nanogels is important for biomedical purpose since interaction with drugs, biomacromolecules or biological agents mainly depends of their functional groups. Spectroscopy results did not evidence considerable changes between spectra of irradiated solutions and polymer spectra, as previous studies.15 It indicates that polymer functional groups are not affected during the nanogel formation and the chemical nanogel structure is similar to the initial polymer (Fig. 2). Therefore, properties such as of drug loading capacity or cell interaction can be influenced by carbonyl, hydroxyl, and amino groups present in the polymer matrix.25
Within the features of nanogel, in order to design successful tissue scaffold, cell culture support or drug delivery system, key points are biocompatibility and immunologic response.1,2
The PVP nanogels are biocompatible since they did not show any toxic effect on Vero cell lines. The cell viability even increases with the nanogel concentration (Fig. 4). This result is remarkable and interesting because in most of cases cell viability decreases when the concentration of a hypothetical compound (including nanostructured ones) increases.34,35 Although this preliminary behaviour could suggest cell protection or antioxidant capacity by nanogels, it is necessary to study deeper, in other cell lines. We recently evaluated the cytotoxic effect of nanogels on murine fibroblast founding similar results.36 Besides, these nanogels are unable to induce massive neutrophil activation (Fig. 5 C) presenting an activation pattern similar to widely used biomaterial TiO2 (Fig. 5 D), in accordance with the literature,37 where titanium particles elicited low inflammatory responses.
In conclusion, PVP nanogels were synthesized by (γ) gamma radiation at room temperature in diluted solutions. The irradiation dose has an important influence on nanogel formation which was obtained at dose value ranging from 10 - 15 kGy. The nanogels have an elliptical shape and their chemical structure preserves PVP functional groups. In vitro assays indicate that this nanomaterial is biocompatible presenting an activation pattern similar to TiO2 demonstrated by a comparative analysis of the NBT test. Therefore, nanogels could be used for biomedical purposes as potential biomaterial, due to their small size, shape, chemical and biological characteristics.