INTRODUCTION
It is well known that the atomic nucleus is a complex many-body system. The knowledge of the internal degrees of freedom is crucial to understand nuclear structure features like single-particle and collective states, clustering, pairing correlations, and other properties [1]. This information canbe extracted from elastic, inelastic, transfer, and other nuclear reactions.Transfer reactions have been extensively studied during the last decades because of their sensitivity to the nuclear structure of the interacting partners.In particular, two-nucleon transfer reactions have gained special attention because they can probe pairing correlations in nuclei [2], [3], [4], [5], [6], [7]. In addition, they are relevant to model more complex processes such as double charge exchange reactions, which are of interest for applications in neutrinoless double beta decay researches [8], [9].
In the last few years, a study of different systems was pursued at the Catania INFN-LNS laboratory (Italy) by the (18O,16O) two-neutron transfer reaction at 84 MeV using the MAGNEX large acceptance magnetic spectrometer to detect the ejectiles. Thanks to its high resolution and large acceptance, high quality inclusive spectra have been obtained, even in a largely unexplored region above the two-neutron emission threshold in the residual nucleus.
New phenomena appeared, such as the dominance of the direct one-step transfer of the two neutrons and the presence of broad resonances at high excitation energy in the 14C and 15C spectra [2],[10]. These structures were recently identified as the first experimental signature of the Giant Pairing Vibration (GPV) [11], [12], [13] predicted long time ago [14].
MATERIALS AND METHODS
The experiments
The experiments were performed at the INFN-LNS laboratory in Catania. The beam of 18O6+, accelerated at 84 MeV incident energy by the Tandem Van de Graaf, impinged on different thin solid targets (namely 9Be,11B, 12C,13C,16O, 28Si and 64Ni targets) produced by evaporation at the LNS chemical laboratory. The beam-integrated charge was measured by a Faraday cup mounted 15 cm downstream of the target. So absolute cross-sections were extracted.
The ejectiles of 16O were momentum analysed by the MAGNEX spectrometer working in the full acceptance mode (solid angle Ω~ 50 msr and momentum range Δp/p~ 24%) [15], [16], [17]. In the different experimental runs, the optical axis of the spectrometer was centered at laboratory angles θopt = 6°, 12°, 18°, 24°. In all the runs the ejectiles trajectory were accepted between -5.2° and +6.3° in the horizontal direction and ± 7.0° in the vertical, with respect to the optical axis. In such a way, an angular range between about 3° and 30° was measured in the laboratory frame with overlaps of about 6° between two contiguous sets of measurements.
The energy spectra
Thanks to the spectrometer high resolution and large acceptance, high quality inclusive spectra were extracted, even in a largely unexplored region above the two-neutron separation energy in the residual nucleus [19],[20],[21],[21], [23], [24], [25].
Examples of the obtained excitation energy E* = Q 0 - Q (where Q 0is the ground to ground state Q-value) spectra are shown in Fig. 1 for different targets and angular settings. For example, in Fig. 1 a) the 14C spectrum at 9° <θ lab < 12° is represented. Several known 14C excited states are populated for which the spin and parity have been determined by previous (t,p) reactions [26]. It is known [27] that the dominant configuration of the ground state and the states at 7.01 and 10.74 MeV is a pair of two neutrons with L = 0, 2 and 4 on a 12C 0+ core, respectively. It is very interesting to note that this spectrum is verysimilar to the ones excited by (t,p) reactions, indicating the strong selectivity of the (18O,16O) reaction. The energy spectrum for the 13C(18O,16O)15C reaction in the angular range 9.5° <θ lab < 10.5° is shown in Fig. 1b). The two bound states of 15C are recognized below the one-neutron separation energy (S n = 1.218 MeV), namely the ground and the state at 0.74MeV. These are characterized by a well-known single-particle configuration with thevalence neutron in the 2s1/2 and 1d5/2 shell over a 14C 0+ ground state core, respectively.Above S n , narrow resonances at excitation energy of E* = 3.10, 4.22 4.66, 6.84 7.35 MeV are clearly identified. Such states are typically labeled as 2particles-3holes configurations and are strongly excited also by the (t,p) reaction reported in Ref. [28] Above the two-neutron emission threshold (S 2n = 9.394 MeV), two large unknown structures are strongly excited at E* = 10.5 and 13.7 MeV over a continuously distributed strength due to the three-body and four-body phase-spaces.A more detailed analysis of the two-neutron transfer on the 15C continuum is reported in Ref. [29].
Another interesting feature of the 14C and 15C spectra in Figure. 1 a) and b) is the appearance of two new structures above the twp-neutron emission threshold at16.9 ± 0.1 MeV and 13.7 ± 0.1 MeV, respectively. By a detailed analysis of these structures, reported in refs. [11], [12], [13], they were recently identified as the first experimental signature of the Giant Pairing Vibration (GPV) predicted long time ago [14].
In Figure. 1c) some results concerning the experiments on 11B target are shown. In ref. [20] a first evidence of pairing correlation was already discussed in presenting the transfer yields. Here we focus on the 13B energy spectra, where several peaks corresponding to transitions to known bound and resonant states and a broad structure between 10 and 14 MeV are observed. In particular, the states at E* = 3.68, 4.13, 5.39,6.16, 6.43 and 8.14 MeV are the most populated. It is interesting to describe 13B lowlyingstates in the limit of a weak coupling among the 3/2- proton hole orbital and the 14C excited states. In this way, a direct link is established between 13B and 14C states at low excitation energy, which are characterized by the correlations of two neutrons in the sdshell. In comparing such homologous states, an interesting finding is the reduction of theenergy of the 13B excited states of about 3 MeV compared to those of 14C. Since the ground state of both nuclei presents a N = 8 magic number of neutrons, due to the p-shell closure, our finding indicates that the energy gap between p and sdshells is lowered from about 6 MeV in 14C to about 3 MeV in 13B. As a consequence the rapid evolution of the shell structure towards 12Be is confirmed, indicating that the N = 8 shell closure is dissolving when neutron-rich nuclei are considered.
An example of 66Ni energy spectrum is shown in Figure. 1 d). In this case the spectrum features are sensibly different from the light nuclei ones. A large bump is observedprobably due to the convolution of the several peaks populated in this region, due to particularly favorable kinematical matching conditions [23]. However, one should notice that for such heavy nuclei the incident energy corresponds to 1.7 times the Coulomb barrier.Thus the dynamical conditions could be rather different compared for example to the 13C target case, where the energy is 3.2 times the Coulomb barrier. Specific analysis based on semi-classical transport equations in heavy-ion collisions have been published in [30].
The angular distribution
The absolute cross section angular distributions of the identified peaks were extracted in a quite wide range at forward angles, according to the procedure described in Ref. [31]. From their analysis it was demonstrated that the (18O,16O) two-neutron transfer reaction can be used for quantitative spectroscopic studies of pair configurations in nuclear states [2], [3], [24], [25], [23], [32]. Examples of angular distributions for the transitions to the 14C ground state and 15C low-lying excited states are shown in Figure 2 and 3.
From a theoretical point of view, the Coupled Reaction Channel (CRC) approach is necessary to interpret such data. In ref. [2] the experimental absolute cross section of the one- and two- neutron transfer reactions induced by an 18O beam on a 12C target was reproduced for the first time without the need of any scaling factor by means of Exact Finite Range (EFR) CRC calculations. Two approaches are shown here in this case: the extreme cluster model (where the two transferred neutrons are treated as a cluster system with the two neutrons coupled with relative spin S = 0) and a sequential two-step approach. The relevance of cluster configurations was revealed in the ground state wave functions of the 14C nucleus, which was described within the extreme cluster approach, see Figure 2. On the other hand, the contribution of the sequential transfer mechanism appears small.
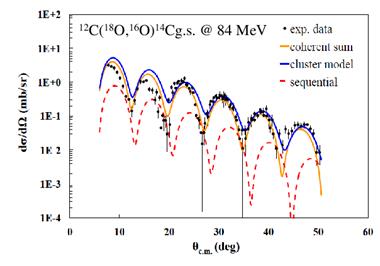
Figure 2 Comparison of the experimental angular distributions with theoretical calculations in extreme cluster model (blue), sequential (red) and coherent sum of the two processes (orange) for the 12C(18O,16O)14Cg.s. reaction.
However, the strong approximation adopted in the extreme cluster approach makes it useful only in few cases, e.g. it does not describe well the higher excitation energy states [2]. In particular, in the case of the extreme cluster model with spectroscopic amplitudes set to 1, often the calculated cross sections are larger than the experimental data. See for example the comparison with experimental data in the case of transitions to the 15C states shown in Figure 3. The main reason for this overestimation might lie in the approximation that the two neutrons are coupled to the total spin S = 0 with 100% of probability. For this reason we developed in Ref. [32] a microscopic cluster model, where spectroscopic amplitudes from shell-model calculations. To achieve this objective, we made use of transformation brackets connecting the wave functions for two particles in an harmonic oscillator common potential (j-j coupling) with the wave functions given in terms of the relative and centre of mass coordinates of the two particles (LS coupling).As a first step, we performed microscopic cluster calculations considering that the cluster relative motion state is represented exclusively by n = 1 and l = 0 quantum numbers, i.e. the cluster is in the 1s intrinsic state. The obtained cross sections (labelled 1s microscopic cluster) are much lower than data for all transitions. Thus, we included also the 1p (n = 1 and l = 1) cluster relative motion states (labelled 1s +1p microscopic cluster). We see in Figure 3 that the 1s + 1pmicroscopic cluster calculations are in rather good agreement with the experimental angular distributions, without the need of any scaling factor.
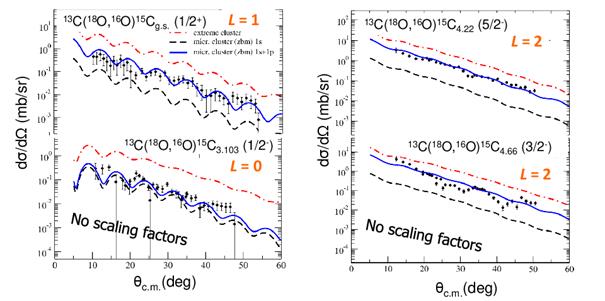
Figure 3 Comparison of the experimental angular distributions with theoretical calculations in extreme cluster model (red), microscopic cluster in the 1s state (black) and microscopic cluster in the 1s + 1p state (blue) for the 13C(18O,16O)15C reaction populating different low-lying states of 15C.
The microscopic cluster modelhas allowed to well describe the experimental cross section, thus demonstrating the importance of a two-neutron correlation in the nuclear wave function in the two-neutron transfer mechanism. A dominance of the 1s and 1p waves in the two-neutron cluster internal wave function is found. This result show that the extra neutron in 13C, when compared to 12C, does not destroy the neutron-neutron correlations in the wave functions.