INTRODUCTION
Science is a human activity that tends to the actual knowledge of things by their principles and causes. Scientific activity constitutes one of the most outstanding achievements of human beings, and it would only be possible if the scientific method (with its particularities depending on the field of study) had gradually developed. Science is a knowledge acquisition system based mainly on this method. Scientific knowledge builds from successes and errors, paths researchers have followed since ancient times, inspired by the work of those who preceded them.1 Scientific progress is, then, inherently linked to the development of culture and often allows the implementation of various technological applications in society, such as biotechnology.
Modern molecular genetics, genetic engineering, current genomics, and, in general, molecular biotechnology with its various applications in health and productive activities would not have been possible if, seventy years ago, in 1953, the structure of deoxyribonucleic acid (DNA) had not been elucidated by James D. Watson, Francis H. C. Crick,2) Maurice Wilkins,3 and Rosalind Franklin4) a discovery for which celebrates its Platinum Anniversary this year.5,6,7) Rarely does a paradigm (i.e., a great framework of ideas that guides investigative knowledge for years) change in science. In this case, it was in the biological sciences. In addition, on rare occasions, we see transcendental models appear, impacting and changing all life and health sciences forever, especially medicine. We have seen these scientific revolutions previously with the discovery of the laws of heredity by Johann Gregor Mendel (forgotten until its rediscovering)8,9) with the Theory of Evolution by Selection Natural by Charles Darwin and Alfred Russel Wallace and, in this case, we also see it with the “Watson and Crick Model”;10) which poses a double helix structure for DNA and whose characteristic is to predict the replication mechanism of genetic material. The elucidation of the double helix structure constitutes one of the great revolutions that originated the field of Modern Molecular Biology,1,6 perhaps the most important scientific advance of the second half of the 20th century.
Therefore, this historical article has the objective to highlight the importance of elucidating the structure of DNA in the celebrations of the seventy years since its discovery.
MATERIALS AND METHODS
The type of study comprised the historiographic methodology. The path to writing this historical article was a traditional mixed narrative review, integrating some chronological and critical evaluative review elements.
The study included searching for articles in databases such as Pubmed, Web of Science and Scopus and articles and books in the academic search engine Google Scholar. The languages used for the search were English, Spanish and French; and the keywords used were “Watson and Crick model”, “discovery”, “DNA structure”, “Rosalind Franklin”, and “double helix”. The search considered articles from the last five years, but a few others considered classics were included, given the historical focus of this work as it focuses on a relevant discovery from the mid-20th century. Of the 109 sources initially found, 62 were freely accessed, and 38 were finally analysed due to their relevance and pertinence to the topic. The information search ranged from January 02 to February 29, 2024.
DEVELOPMENT
In the search for a structure
Cell biology (formerly called Cytology) had a gradual emergence, entirely conditioned by the advances in microscopy due to the gradual development of light microscopes, which became increasingly sophisticated.11) At the beginning of the second half of the 19th century, it was gradually possible to understand the intimate structure of cells and their organelles, but not with the ultrastructural detail that modern electron microscopy offers us today, whose first microscopes appeared in the 1930s.11
Knowledge of nucleic acids had an initial impulse when Friedrich Miescher, a Swiss biochemist and physician, discovered “nuclein” in 1869, the original name given to nucleic acids,12 but little was known about this substance. For several decades, its role was not linked to genetic information transmission. Furthermore, most researchers in the area thought that the transmission of hereditary characters was a function of proteins, understanding that these are more complex, structurally speaking, than nucleic acids. It wasn't easy to accept that genes (transmitters of hereditary information) resided in DNA, a simpler molecule. Hence, the mainstream scientific community resisted the idea that such complex information is stored in DNA. For this reason, the research community continued to consider proteins as the fundamental biomolecules in transmitting hereditary characteristics.6
It was not until Oswald Avery, Colin McLeod, and Maclyn McCarty carried out their classic experiments on pneumococci13) that DNA began to be identified as the carrier of genetic information (of the “transforming principle”). However, the coup de grâce that confirmed this was Alfred Hershey and Martha Chase's elegant experiment,14) who, using radioactive isotopes in their work with the T2 phage, demonstrated that the transforming principle was DNA. In the intense race to elucidate the structure of DNA, the prominent chemist Linus Pauling, considered by Watson the best chemist in the world, actively participated.12,15) For years, he had worked with his team on protein structures and verified with crystallographic techniques that the secondary structure of proteins could be of two types: the beta-sheet and the alpha helix.16) In parallel, since the 1940s, chemist Erwin Chargaff and his team have dedicated themselves to investigating the composition of nucleic acids in various species, particularly concerning the purine (adenine and guanine) and pyrimidine nitrogenous bases (cytosine and thymine). Through experiments by Chargaff and his team, it was found that the relative proportions of the four nitrogenous bases were not random.17) The amount of adenine in all DNA samples studied was equal to that of thymine, whereas the amount of guanine was equal to that of cytosine.18) Furthermore, regardless of the origin of the DNA, the purine to pyrimidine bases were always close to the 1:1 ratio (Chargaff’s rules).17) Thus, the structure of DNA was still a great unknown, but its stoichiometry had already been defined. One year later, Pauling and Corey19 proposed a novel but mistaken triple helix structure for DNA. Nevertheless, the solution to the problem of the mysterious structure of DNA, considered the holy grail of biology, finally came in 1953 from the American biologist James D. Watson and the British biophysicist Francis H. C. Crick and other researchers.1,2,3) Using the composition of nitrogenous bases determined by Chargaff as well as X-ray diffraction photographs of DNA taken by Rosalind Franklin (a brilliant and very skilled crystallographer4,20) who worked with the biophysicist Maurice Wilkins), Watson and Crick proposed their structure for DNA,2) constituting a transcendental scientific contribution that allowed counting with the Rosetta Stone to decipher the encoding of genetic information further. This finding triggered the entire development of modern Molecular Biology, taking it to the zenith of its golden age.1,6
The studies by Watson, Crick and other researchers (Figure 1A) made it possible to establish that the DNA molecule comprises two polynucleotide antiparallel chains or strands wound one over the other, constituting a double helix, like a spiral staircase. On the outside of the helix are the skeletons of the chains made up of sugars (deoxyriboses) and phosphates, and on the inside are the purine and pyrimidine bases. A series of weak interactions (hydrogen bonds) establishes between these bases, the sum of which gives the structure great firmness. (Figure 1B) The bases, as mentioned, pair according to Chargaff’s rule: adenine with thymine and cytosine with guanine. Thus, the essential simplicity of the DNA structure was consistent with the observation by Chargaff that in all DNA, the molar content of adenine equals that of thymine, and the molar content of guanine equals that of cytosine. Although some model details have been modified, the Watson and Crick hypothesis was quickly accepted, and its essential elements have remained unchanged from their original proposal.10) Due to the importance to humankind regarding their contributions, Watson, Crick, and Wilkins received the Nobel Prize in Physiology or Medicine in 1962, specifically for their discoveries on the molecular structure of nucleic acids and their importance for the transfer of information in the living matter. The skilled chemist Rosalind Franklin did not receive the Nobel Prize because she died of ovarian cancer in 1958.
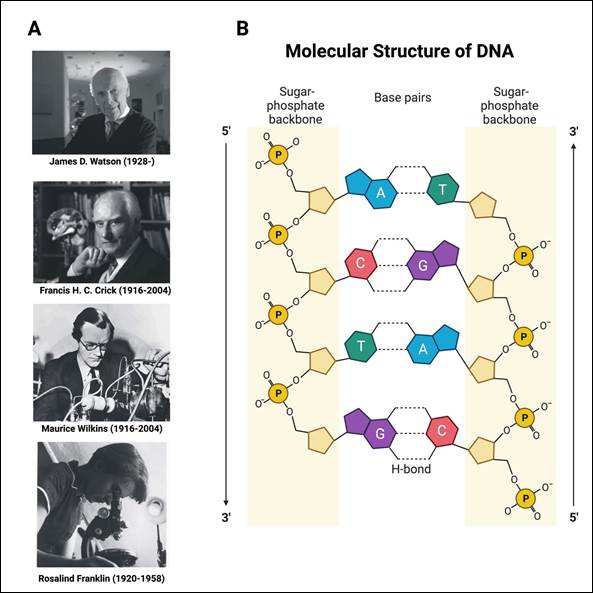
Figure 1 In the scientific race to solve the mystery of the molecular structure of DNA, the work of several scientists converged. This finding included various theoretical predictions and experimental approaches. Main scientists involved in elucidating DNA structure (many other leading scientists are involved, but due to space issues, they were not included in this figure)
The discovery of the DNA structure in 1953 by Watson and Crick undoubtedly revolutionised the field of Molecular Biology.7) It led to significant advances in our understanding of Genetics,21) not only of this field but of the entire phenomenon of life with all that it implies, even from the philosophical point of view.15,16) Countless subsequent experimental findings confirmed the “Watson and Crick model” validity. One of the most important attributes is its ability to predict a replication mechanism of genetic material. Each DNA strand is complementary to the other strand because, according to Chargaff’s rules, if the base sequence of one of them is known, that of the other is automatically determined. A crucial concept in molecular biology arose from this complementarity, the template concept: a DNA strand serves as a template for the synthesis of its complementary strand. The work of Watson and Crick solved a novel biochemistry and biology puzzle. It simultaneously catalysed the emergence of a new paradigm as the convergence of two lines of research, one on the shape of the molecule of life, the other on its hereditary function.16) Although, on the one hand, in simple terms, DNA is a macromolecule consisting of two spirally twisted chains that store and transmit hereditary information, constituting a genuine “hard disc” for life information22) one way or another, it is also memory, the repository of information that our ancestors transmitted to us. Hence, this nucleic acid defines our past and future. The resolution of the DNA structure allowed access to one of the secrets of life. The famous publication of 1953 inNature2 on the elucidation of the structure of DNA as a vehicle of heredity led to an explosion of knowledge in the area of Molecular Biology, validating the view that living beings are highly organised systems composed of cells that, in turn, are composed of molecules and the fruits of a long process of evolution.
Since the establishment of DNA structure, significant progress has been made in Molecular Biology and the more specialised field of Molecular Genetics. It would be impossible to summarise them all, but among them stands out the approach of the Central Dogma of Molecular Biology by Francis Crick,23) a revolutionary theory for biological sciences that postulates that genetic information flows in only one direction, from DNA to RNA and from this to protein, or from RNA (ribonucleic acid) directly to protein. However, the Dogma was not an absolute. In 1970, American researchers Howard Temin and David Baltimore independently demonstrated that the flow of information from DNA to RNA was not strictly unidirectional. For example, some viruses have a reverse transcriptase enzyme capable of copying DNA using RNA as a template.24 These viruses, known as retroviruses, are relevant to health since they are responsible for AIDS and certain types of cancer.24 Both scientists received the Nobel Prize in Physiology or Medicine in 1975 for this discovery. Another enzyme that has this reverse action is telomerase, which is of great relevance in the synthesis of chromosome ends (telomeres) and whose action is altered in cancer cells.24
Biomedicine undoubtedly made progress thanks to the discovery of the DNA molecule seventy years ago. Mastery of recombinant DNA technology during the 1970s allowed scientists to manipulate DNA and access precise gene analysis.25) The discovery of the double helix structure model of DNA led to the rise of Biotechnology with Genetic Engineering at its core.26 This discovery allowed the development of genetically engineered drugs. The human somatostatin hormone was first produced by American researchers using the bacterium Escherichia coli in 1977. This advance was the first success of genetic engineering research that caused shock worldwide and clarified the theoretical basis of gene expression in higher organisms.26 The enormous economic value was also tempting for further developing the Biotechnology Economy. In 1979, American researchers announced the first genetically engineered expression of human growth hormone. This hormone is specific and expensive, but the genetically modified version is cheap and practical.26 The DNA double helix’s structure discovery also enabled gene therapy development. It does not matter whether it is a genetic, cardiovascular, immune, infectious disease or a malignant tumour; if this disease is related to the mutation, deletion, and abnormal expression of genes, what is required is to create a “normal gene” to replace those “abnormal genes,” find a way to shut down those out-of-control genes, which is called Gene Therapy.26 Gene therapy has shown broad potential in treating various diseases for which no ideal treatment exists. It will become one of the routine treatment methods in clinical medicine in the future.26 The discovery of DNA structure has also made it possible to use plants and animals as bioreactors to produce valuable drugs.26 Transgenic plants can be used as bioreactors to produce vaccines, medicines, and industrial raw materials, which constitutes a new trend in the application of genetic engineering in agriculture and industries of the 21st century. Using genetically modified plants to produce vaccines, medicines and nutrition can improve human and animal health, produce industrial raw materials, and help address environmental problems.26
At the end of the 1980s, some researchers proposed deciphering the whole human genome. Here are the origins of Genomics, which studies genes, their functions, and their interactions in the genome.25,27 However, the first sequenced genome was not the whole human genome due to knowledge and technical limitations in those years and the high costs of obtaining DNA sequences.25,27 Sequencing started with relatively small genomes, i.e., less than 20,000 nucleotides in length. In the 1980s, the genomes of organelles such as mitochondria, chloroplasts, and some viruses were sequenced.25,27 In the 1990s, it was possible to sequence the complete genome of some prokaryotes and brewer's yeast (Saccharomyces cerevisiae), a relatively simple eukaryote.25,27 The Human Genome Project began in 1990 and was completed in 2003. Its objective was to sequence the entire human genome.25,27 Although this project represented a significant advance in understanding the human genome and its complexity, several questions still needed to be answered. The finding that only about 20,000 genes are protein- and RNA-coding is one of the most surprising results of the Human Genome Project. The human genome is as complex and unique as other organisms. These findings demystified the heightened expectations created around human DNA.25,28 There is also no doubt that the Postgenomic Era was initiated thanks to the completion of human genome sequencing in 2003.25,28,29) The information obtained by Genomics advances has been crucial for the new understanding of health and disease from the molecular diagnosis point of view and for developing predictive medicine. Thus, thanks to Genomics, the understanding of the biology of disease and health has increased in an unsuspected way until a couple of decades ago. Therefore, Genomics has revolutionarily modified the practice of modern medicine.30
Significant advances in biomedicine will be achieved from now on if we discover where the complexity of the genome lies.9) This complexity is undoubtedly in the interaction and regulatory networks between genes and gene products. The plethora of information that sequencing of the human genome has yielded can sometimes be stifling to the point of overwhelming, and converting the information we have about the human genome into knowledge will take many generations of researchers. With the advancement of genetic sequencing techniques, we are protagonists of a relevant stage for biomedicine.30 It is increasingly possible to study more of the human genome in less time and at a lower cost, opening the window of opportunities for gene therapy for diseases that have historically not been treated.30 Other advances came from Genetic Statistics, which seeks to develop computational, statistical, and probabilistic methods that allow efficient analysis of genetic data to extract relevant information for medicine and evolutionary population genetics. Given the significant interest in personalised medicine, which seeks to identify through individual genes what would be the appropriate treatment for each one, further development in this area is essential. In the second decade of the 21st century, researchers are now trying to reveal all the secrets the human genome still hides.( 25)
From the information mentioned above, it can be stated that the elucidation of the structure of DNA seventy years ago has been a transcendental scientific fact for the development of humanity.6) Without detracting from their due credit, while Watson and Crick are mostly given credit for this discovery, it is worth mentioning that this discovery constitutes a convergence of the contributions of many other scientists.15) In particular, the contribution of Rosalind Franklin should be remembered. She has become a role model for women interested in science, technology, engineering, and medicine (STEM).4,20,31 It is impossible to know whether Franklin, independently of Watson and Crick, would have understood how the structure of DNA could specify proteins.4,20,31 However, considering her critical role in solving the structure of DNA, the fact that she did not receive adequate recognition in her lifetime continues to generate controversy until now.18,20
The double helix proposal constitutes a paradigm shift in science, which has impacted medicine and the most diverse areas of knowledge. The double helix also constitutes a model that explains and integrates, on the one hand, the experimental data on the structure of DNA and, on the other hand, suggests a mechanism for its replication.18) This fact is the scope of validity of the model. The discovery of the DNA structure catalysed the rise of modern Molecular Biology, revolutionised Molecular Genetics, and, as mentioned, paved the way for the development of Genomics.6,18,25 In addition, from a philosophical point of view, having deciphered the macromolecule structure that stores and transmits genetic information has undoubtedly contributed to our understanding of the phenomenon of life and our place in it.6) Nevertheless, believing that biology has the ultimate key to explaining all our individual and collective behaviour would be to sink into a dangerous “biologism”.32)
The endless curiosity and search for knowledge that characterises human beings will be the engine that will drive scientists to solve new questions about the phenomenon of life during the 21st century. Furthermore, in particular, the study of the complex interrelationships in which the human genome is involved will be a relevant component of the Fifth Industrial Revolution,33 which is impacting all productive areas and whose characteristic of personalisation, exemplified in personalised medicine, will necessarily involve deeper insights into molecular genetics, clinical genetics, and the human genome.29) One of the main characteristics of this Fifth Industrial Revolution is Artificial Intelligence. Artificial intelligence has enormous potential to advance the precision treatment of genetic diseases in genetic clinical care and can be complemented by machine learning applied to Genetics.34 In Genomics, multiple traditional machine-learning approaches are already used to understand the dynamics of genetic data.35 Decoding the human genome using machine learning represents a remarkable advance in Genomics and has opened unprecedented avenues in healthcare and biomedical research.36 The intricate structure of the human genome, composed of chromosomes, DNA molecules, genes, and non-coding regions, still holds secrets from life itself. By using artificial intelligence tools and machine learning algorithms, researchers can effectively analyse and interpret a large amount of genomic data, providing insight into gene function, associations with diseases, and the discovery of targets for new drugs; this, from the point of view of personalised medicine.36 Integrating machine learning with genomics research fosters advances in understanding complex biological processes such as gene regulation, epigenomics, and non-coding RNA functions.36 Synthetic Biology, broadening from inserting artificially synthesised DNA into biological systems to achieve specific functions to integrating biological and non-biological systems and how to use these systems for medical and environmental applications37) will require a deeper understanding of the human genome. Seventy years after deciphering the molecular structure of DNA, we are facing a revolution in genome editing.6,38 Sequencing and synthesising DNA is cheaper and faster than ever, leading to the rapid advancement of molecular biology and biotechnology.38) DNA editing and engineering tools developments advanced our fundamental biological understanding, allowing us to develop societally relevant applications.38) Research will continue in this field, with DNA being one of the main protagonists.
CONCLUSIONS
In conclusion, solving the DNA structure, equivalent to unravelling one of the “secrets of life”, had a profound impact on biological and health sciences, currently changing the way of addressing various genetic issues in biomedicine. Thanks to the discovery of DNA structure, the foundations were laid for human genome sequencing twenty years ago, which meant the beginning of the Postgenomic Era. Reflecting on this transcendental scientific advance is relevant for health professionals and educators interested in the Biomedical Humanities and the History of Medicine since this knowledge is valuable enough to be transmitted to the new generation of in-training health professionals and researchers.