Livestock in Cuba is a strategic objective for dairy production, where the first link is related to the sustainable management of land (Cuella et al.2015), followed by the use of improved grasses, in grazing or in association with forage trees, timber and others, that cover at least 80 % of the energy requirements of animals in a semi-intensive production system (Milera et al. 2018) that contributes to the increase of milk production. But ensuring a quality and safe product is also an interest of producers and the Ministerio de la Agricultura.
The safety of milk is mainly associated with the presence of antibiotics (Panigrahi et al. 2017) and pesticides (Gao and Sun 2018). However, it is increasingly important to analyze other persistent organic compounds (POCs) and inorganic in the food chain (Cuesta et al. 2017). Among the POCs are the polycyclic aromatic hydrocarbons (PAHs), due to the toxic and carcinogenic effects they have, the US Environmental Agency (EPA) has showed a list of the 16 PAHs that should be studied (Li and Ma 2016).
The presence of PAHs in the atmosphere has been reported in Africa, Australia, the Pacific, Europe, Asia, Latin and North America and even in the Arctic, in a range of 1 to 7281 pg/m3 (Bogdal et al. 2013). These can be deposited in the soil and in the plants, so in those places where lactation animals may appear residues of PAHs in milk (Grova et al. 2005 and Costera et al. 2010).
In Latin America, studies on PAHs in grass and milk are limited. It can mention the research carried out on commercialized milks from Argentina and Brazil (García-Londoño et al. 2013) and in grasses and milks from agricultural areas close to petrogenic contaminating sources in the Veracruz, Mexico (Pérez 2012).
In Cuba there is no previous information about the presence of PAHs in grass and milk that allows evaluating the impact of these products in their safety. The objective of this study was to evaluate the concentration of PAHs in grass and milk in livestock areas and the relation between their concentration in food and those reported in the soil.
Materials and Methods
Location of the study area. Previously, a monitoring network was carried out in the Mayabeque and Havana soils, where 69 sites were studied for the analysis of PAHs in soils (Sosa et al. 2017). Of them a total of 25 resulted to be breeders and of them 15 sites were selected (1, 3, 11, 15, 16, 17, 23, 26, 31, 32, 34, 38, 52 and 59). For its selection, the proximity to the polluting sources and the PAHs concentrations in the soils were considered (Sosa et al. 2017). In these sites the grass species that were present were the star grass (Cynodon nlemfuensis), n=9 and guinea (Megathrysus maximus), n=6. Of the 15 sites selected to evaluate the grass, in 10 sites had milk producer cattle (1, 3, 11, 15, 28, 31, 32, 38, 52 and 59) of which one corresponds to buffalo cattle (water buffalo). The cows of the evaluated sites were characterized as being of Creole breed, Siboney de Cuba, zebu and crossbreeding (figure 1).
Sampling, processing and analysis of polycyclic aromatic hydrocarbons from samples of grass and milk. The samples of grass and soil were taken in the same quadrant of 100 m2 (Sosa et al. 2017) with the objective of finding relations about the contamination between both matrices of the agroecosystem. A 1 kg sample was taken, packed in polyethylene nylon envelopes covered with aluminum foil and sealed with Teflon tape, and stored at 4 °C until laboratory analysis (Nadal et al. 2004). The grass was dried at 40 °C until constant weight, subsequently ground and passed through a sieve with an opening of 2 mm. Next, the sample was divided by the quartering procedure and the samples were taken diagonally, for analysis. Sampling was done in the dry season (March, 2016, December, 2016 and January, 2017), since according to Cabrerizo et al. (2011) and Rychen et al. (2008) is the time of highest concentration of pollutants in the grass and soil.
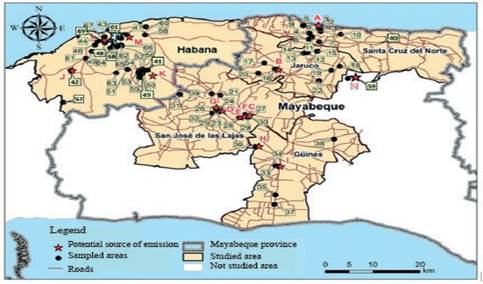
Figure 1 Research area within Havana and Mayabeque
Samples of 500 mL of milk were collected from the collector tank of each dairy in the same period in which the grass samplings were made, as established by the Federación Internacional de Lechería (FIL/IDF 1995) and the general sampling guidelines established by the Codex Alimentarius (CODEX 2004). Once the milk arrived at the laboratory, the fat percentage was determined by infrared spectrophotometry using the Milko-Scan model Minor-6, A/S (Foss Electric, Denmark) (FIL/IDF 141C: 2000) with an accuracy lower than 1.5 %. This equipment was validated and fitted according to ISO 8196-2 (2009). The milk was stored at -20°C and the extraction of fat was done before a week of storage, which is the recommended time for the technique reliability (Grova et al. 2000).
For the analysis of PAHs, the 16 PAHs taken into account by the Environmental Protection Agency were used: naphthalene (NAP), acenaphthylene (ANY), acenaphthene (ACE), fluorene (FLU), phenanthrene (PHE), anthracene (ANT) ), fluoranthene (FLT), pyrene (PYR), benzo [a] anthracene (BaA), chrysene (CHR), benzo [b] fluoranthene (BbF), benzo [k] fluoranthene (BkF), benzo [a] pyrene ( BaP), indene [1,2,3- cd] pyrene (IPY), dibenzo [a,h] anthracene (DBA), and benzo [ghi] perylene (BPE) from the commercial house Promochem (Wesel, Germany). The deuterated compounds of PAHs (d8-NAP, d8-ANY, d10-ANA, d10-FLU, d10-PHE, d10-ANT, d10-FLT, d10-PYR, d12-BaA, d12-CHR, d12-BbF, d12-BkF, d12-BaP, d12-IPY, d14-DBA and d12-BPE) were obtained from the Cambridge isotope laboratory (Andover, MA, USA).The Indeno [1,2,3-cd] -fluoranthene (99.7 % purity) comes from the commercial house Promochem (Wesel, Germany). The rest of the reagents used in the analytical technics are of high quality from well- known commercial companies such as Fluka, Merck and Schleicher & Schuell (Bucheli et al. 2004).
For the extraction, purification and identification of PAHs in the grass samples, 5 g were weighed and transferred to a thimble for extraction by soxhlet, after a solution of internal recovery standard of 20 μg/kg of the 16 deuterated PAHS was added, subsequently was extracted for 36 h with n-hexane and concentrated to 1-2 mL. The concentrated extract was purified by partition and column purification for further analysis by gas chromatography (Agilent 6890) (Gubler et al. 2015 and Bucheli et al. 2004).
In the case of milk, 150 mL were taken and a liquid-liquid partition was carried out in the following order: 1) 150 mL methanol, 2) 150 mL diethyl ether and 3) 150 mL petroleum ether. The obtained extract was rotoevaporated almost to dryness. Then the fat content was determined by gravimetry (AOAC 2005). A column purification of silica and a selective extraction with n-hexane (F1) were carried out; hexane: dichloromethane (9: 1 v/v) and n-hexane: dichloromethane (1:1 v/v) (F2) (Gutiérrez et al. 2015). The detection and quantification was performed by gas/ mass chromatography (Bucheli et al. 2004).
Parameters of the performance of analytical technique to determine polycyclic aromatic hydrocarbons (PAHs) in grasses and milk. The quantification of PAHs was performed by the internal standard method with their respective labeled compounds (Bucheli et al. 2004).
Grasses: The calibration curve was linear showing a regression coefficient higher than 0.99 for the PAHs. The detection limit (DL) and quantification limit (QL) of individual PAHs ranged between 0.01 and 0.12 μg/kg and 0.04 and 0.39 μg/kg, respectively. The recoveries of the native PAHs added were higher than 83 % (five replications containing 40 μg/kg of the 16 deuterated PAHs). The corresponding coefficients of variation of the individual analytes for the repeatability and reproducibility study ranged between 4.5-23 % and 5.5-23 %, respectively in all cases the Horrat index was less than 2. All the results were in accordance with the Nordic Standard (NMKL 2010).
Milk: The calibration curve was linear showing a regression coefficient higher than 0.99 for the PAHs. The detection limit of methods (DL) and quantification (QL) of the individual PAHs ranged between 0.36 and 2.34 ng/mL and 1.9 and 7.5 ng/mL, respectively. The recoveries of the native PAHs are above 80 % (five replications containing 800 μg/kg of the 16 deuterated PAHs). The corresponding variation coefficients of the individual analytes for the repeatability and reproducibility study ranged between 2.65-12.66 % and 3.60-22.68 %, respectively in all cases the Horrat index was lower than 2. All the parameters are in accordance with the specifications of the Nordic validation standard (NMKL 2010).
Statistical analysis. Linear regression analysis was performed with the use of Excel Office 2013. Descriptive statistics and ANOVA analysis were applied with Tukey’s multiple means comparison method (Miller 1981), carried out with the InfoStat program version 2012 (Di Rienzo et al. 2012).
Results and Discussion
The sum of the 16 PAHs in the selected grass samples ranged between 29 and 157 μg/kg (mean 67 μg/kg, median 45 μg/kg). The mean value of the PAHs in the evaluated grasses is in the range of the contamination that has been reported in grasses (25-900μg/kg) and is lower than the one reported (142-3989 μg/kg) in industrialized areas (Crépineau et al. 2003, Grova et al. 2000 and Rychen et al. 2008). This corresponds to the location of the studied sites, since they were all in rural areas (Mayabeque); except site 52, which despite being considered as an urban site (Cotorro, Havana) showed similar concentrations to the rest.
The PAHs of 2 to 3 rings presented a range of 0.58-14.04 μg/kg; those with 4 rings of 1.55- 5.83 μg/ kg and those with 5 to 6 rings of 0.14- 3.84 μg/kg. As the sum of the 16 PAHs increases, the sum of PAHs of 2-3 rings, 4 rings and 5-6 rings increases, but in the case of PAHs with 2 to 3 rings and 4 rings it is much more pronounced ( figure 2). This corresponds to the PAHs that most contributed to the total sum: NAP (11 %), PHE (30 %), FLT (10 %), PYR (10 %) (figure 3). This performance is in agreement with other reports, in which PAHs have predominated such as: FLT, PYR and PHE (Crépineau et al. 2003 and Grova et al. 2000). It has been reported that the PAH-BPM adhere to the intracuticular wax of the plant and those of high molecular weight to the epicuticular wax and are therefore are more exposed to photodegradation and washing, so it is common that in the leaves of the plants tend to abound the HAP-BPM (Bakker et al. 2001).
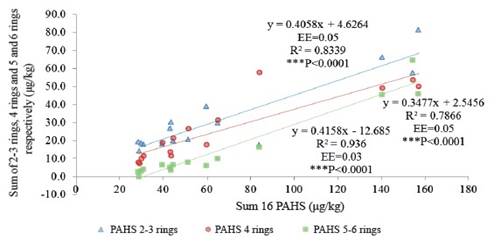
Figure 2 Performance of polycyclic aromatic hydrocarbons (PHHs) in the grass vs. the sum of PAHs of 2-3; 4; 5-6 rings.
Only when the contaminating source is powerful, constant and the grasses are very close to it (distance lower than 50 m), the tendency to predominance of PAHs of high molecular weight (PAHS-HMW) can be observed. On the other hand, if they are far from this, the PAHs-LMW will be abundant (Crépinau et al. 2003). In our case, the sampling sites were found more than 50 m from the contaminating sources, so the observed performance is logical. Only one site had a different performance (site 23 near the cable factory) and it is because it is very close to the contaminating source (figure 1). It should be noted that, although they do not predominate, in the studied grasses, there is PAH-HMW, which indicates the influence of industrial sources or the emission of vehicles. In addition, it has been reported that grasses from rural areas that have little influence from the emitting sources do not have PAHS-HMW, but only PAHS-LMW (Tankari et al. 2007). It should be highlighted that there is no legislation for PAHs in grass at the international level and therefore, Cuba does not have regulations in this regard, hence the importance of having preliminary results that allow regulators to know the current problems of each area.
Figure 4 (a and b) shows the molecular relations of BaA/(BaA + CHRY) vs FLU/(FLU + PYR) and IPY/(IPY + BPE) vs. FLU/(FLU + PYR), respectively, as established by Buchelli et al. (2004) to indicate the origin of the contamination. The sites 16, 23 and 38 have a molecular relation that shows that the contamination is from the petroleum combustion, which is related to emissions from vehicles and industries. In the rest of the sampled places the pollution comes from the burning of wood, grass or carbon. In the case of the BaA/(BaA + CHRY) relation there are 4 sites that denote that the source of contamination is by combustion (4, 12, 32 and 38), the first two are under the influence of industrial action, the third by the biomass burning and the fourth by traffic. In the other evaluated grasses, the origin of the contamination is mixed (pyrogenic and petrogenic). The contamination is by combustion in the IPY/ IPY + BPE) ratio in sites 16, 17, 23 and 28, which receive emissions from vehicles and industries. It can be summarized that the contamination in the grasses is of pyrogenic origin (combustion) and by mixed sources (pyrogenic and petrogenic).
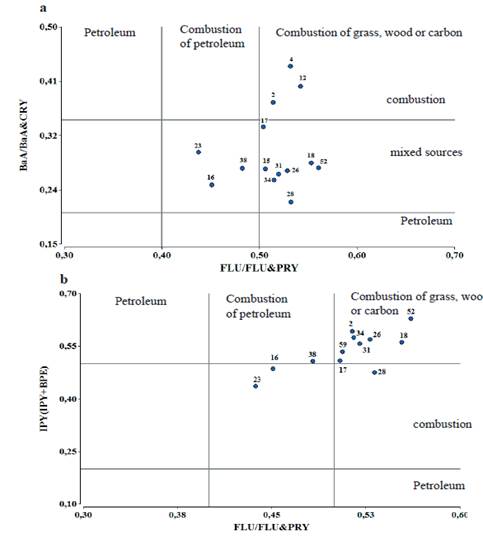
Figure 4 Relations of the polycyclic aromatic hydrocarbons (PAHs) that indicate possible origins of the contamination in the grasses. (a) BaA / (BaA + CHRY) vs FLU/(FLU + PYR); (b) IPY / (IPY + BPE).
Although there is no significant difference between the concentration of the 16 PAHs of the grasses and the soils of the 15 studied sites (P> 0.05), it can be observed that in 53 % of the samples the relation is higher than 1 and in 47 % lower than 1. Since 1983, it has been reported that the concentration of PAHs is higher in the soil than in the plants that are grown in it. However, in a study in the tropical region of China (Shunde) it was found that there was a relation in the concentration of PAHs between the plant and the soil of 0.53-4.23 with a mean of 2.2 (Li et al. 2008). In our case, the range was between 0.13-3.02, with an average of 1.3, there being no significant correlation between them (y = -0.009 + 67.65, SE= 0.11, R2 = 0.0005, P> 0.05). This effect of bioconcentration and poor correlation can be explained by the high atmospheric deposition and the loss of PAHs in tropical soils. The factors that can influence that there is a lower concentration of these compounds are: high temperatures, higher microbial degradation, photo-oxidation, volatilization and leachate (Wikle et al. 1999).
When two groups according to the value of the relation between the concentration of the 16 PAHS between the grass (dependent variable) and the soil (independent variable) (> 0 < than1) were made a positive and significant correlation was observed. The sites which showed a value of the ratio higher than 1, which indicates that the atmospheric deposition predominates (y =2.4109x -11.073, R² = 0.8444, SE=0.42, P<0.01), and the sites that had a ratio lower than 1 (y = 0.0682x + 30.284, R² = 0.7388, SE=0.02, P≤0.05).The strong associations between the variables showed that the PAHs concentrations in the soil influences on the grass.
In this research in the studied sites two grass species predominates: star (Cynodon nlemfuensis) and guinea (Megathyrsus maximus).These two species have different botanical characteristics. The surface of the star grass leaves is semi-succulent, with smooth margin and medium and long size. On the other hand, the guinea grass is characterized by having long and wide leaves, very well distributed in the stems, with a high ratio of leaf/stem and regrowth rate.
Each plant species has different levels of long chain hydrocarbons (n-alkane) that are part of the cuticular wax, so they have their own “fingerprint” (Cesa and Bakker 2007). Higher n-alkane values are reported in the literature for the species Panicum maximum (385 mg/kg of dw) in relation to Cynodon nlemfuensis (213.7 mg/kg of dw) (Molina et al. 2004), therefore, it was expected to find a difference in the concentration of PAHs in the analyzed species (Srogi 2007). Aspect that was not evident, not finding significant difference with respect to the 16 PAHs, nor in the majority compound (PHE) in both species. It must also taking into account that these analyzes were carried out in real conditions, and the contamination is multifactorial, the sites were in different anthropogenic activities, which could have influenced on obtained result.
There are few studies related to the phytotoxic power of PAHs. It has been shown that the presence of PHE in soil and in two plant species significantly induced a lower development of new biomass shoots (Desalme et al. 2011).
It can be thought that the predominance of PHE in the soil in our climatic conditions can affect the yields of the grasslands, an aspect that has not been studied and is of great importance in the livestock feeding strategy at present. If it is considered that in the tropics it is impossible to aspire to sustainable milk or meat production without the use of grasses and forages as the main livestock food base. The results of this study constitute a precedent for future researches in which different experimental designs can be performed.
The concentration values of PAHs in milk ranged from 6.86 to 13.49 μg/kg of milk, with a mean and median of 10.24 μg/kg and 10.22 μg/kg, respectively. Only PAHs with 2 to 4 rings were detected and PHE (56 %) and FLU (21 %) predominated (figure 5).
The obtained results are in the same order of magnitude than those reported by other authors (Aguinaba et al. 2007 and Naccari et al. 2011) (where the individual values of PAHs are compared). However, they are two orders of magnitude lower than the concentrations found in milk from industrial zones in Hidalgo, Mexico (Gutiérrez et al. 2015). Studies have shown that in milk the PAH-BPM are excreted with more easily and more quantity, such as PHE and PYR, and those of high molecular weight such as BaP are excreted mainly in faeces (Lapole et al. 2007 and Grova et al. 2005). In our study only HAP-BPM were detected, which is in accordance with the theory that the absorption of PAHs decreases with the increase of molecular weight (Grova et al. 2005), in addition to these milks come, fundamentally, of rural areas with the action of few contaminating sources. Similar results in relation to the presence of PAHs in milk were found in a study in rural areas, while in milks from urban areas near the emission sources in France, appear HAP-APM such as the BaA and CHR (Grova et al. 2000).
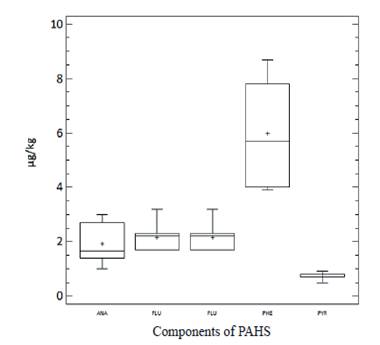
Figure 5 Policyclyc aromatic hydrocarbons (PAHs) presents in the milk from livestock areas of Mayabeque province and Site # 52 of Havana
At present not only attention is paid to native PAHs, but also the biotransformation process of these ones in the organism is taken into account; where the hydrolyzed metabolites of PHE and PYR (1-OH- PYR and 3-OH-PHE) and their native compounds appear at a higher rate than the hydrolysed metabolite of BaP (3-OH-BaP) (Lapole et al. 2007). On the other hand, the secondary metabolites have a 10 times higher excretion than their original compounds (Jurjanz et al. 2008).
In this study it was observed that as the sum of the 16 PAHs increases, the concentration of PHE, FLU and PYR increases (figure 6). The PHE is the one with the highest concentration as the 16 PAHs increase. This is due to the fact that the PHE easily dissolves in an aqueous medium (rumen), from leaves with a wax surface (example: grass), rapidly metabolizing (Costera et al. 2010) and excreting in milk (Grova et al. 2005).
The scientific opinion of the Panel of Contaminants in the Food Chain (EFSA 2008) determined that the BaP alone is not an indicator of the occurrence of PAHs in food and have established as markers the set of 2 PAHs (BaP + CHR), 4 PAHs (2 PAH + BaA + BbF) and 8 PAHs (4 PAH + BkF + BPE + DBA+ IPY). In August 2011, the current regulation 835/2011 (UE 2011) was established, which became effective on September 1, 2012 and established the maximum permissible limits of these compounds at 1.00 μg/kg of BaP and the sum of the 4 PAHs (CHR, BaA, BaP, BbF) in fat and foods with fat such as milk (Purcaro et al. 2013).
A study conducted on milk marketed in Argentina and Brazil reported correlations between 2 PAHs and 4 PAHs of 0.95 (even higher than those obtained by EFSA), between 2 PAHs and 8 PAHs of 0.71 and between 4 PAHs and 8 PAHs of 0.83 (García-Londoño et al. 2013). In our case, high molecular weight compounds were not found, so the respective correlations were not established, being necessary to emphasize that the milks analyzed have a lower risk for human health. But these data are of great importance because PAH-LMW are barely legislated in food globally and there is a regulation in Germany that has paid attention to them. It has established food limits for PAH-HMW (> 5 rings) of 5 mg/kg and for the total content of PAH-LMW (<3 and 4 rings) of 25 mg/kg of oil or fat (Barra et al. 2007). Canadian legislation only covers PAH-HMW (maximum limit, 3 mg/kg) (BaP, DBA, BaA, BbF, BkF, CHR, and BPE), calculated on the basis of the equivalent toxic factor (TEF) (Gutiérrez et al. 2015). The concentrations detected in this study also do not exceed these values.
In this research the presence of the 16 PAHs was observed (except in milk, which was not reported the NAP and the PAH-HMW were not observed) in the three matrices: soil (Sosa et al. 2017), grass and milk. Although it is very difficult to find correlations between them because the contamination is multifactorial; it was observed in sites 1, 3, 11 that they had in common the contamination source (thermoelectric, SC) a good significant correlation between the concentration of PAHs in the grass(independent variable) and in milk (dependent variable) (y = 0.1848x-17.675, SE = 3.9E-03, R2 = 0.9996, p <0.05).
Conclusions
The presence of PAHs was characterized in grasses and milk, fundamentally from Mayabeque province. It is considered that these foods have a low risk to animal and human health, where milk is considered safe according to European regulations for PAHs. There is a relation in the concentrations of PAHs between soil, grass and milk. This study constitutes the first antecedent that guides that the PAH-LMW are the ones that predominate in grasses and analyzed milks, being the PHE the compound that is in greater proportion.