Introduction
Ketoprofen is a potent nonsteroidal anti-inflammatory drug (NSAID) of the propionic acid derivative group with analgesic and antipyretic properties. Ketoprofen has pharmacologic actions similar to those of other prototypical NSAIDs that are thought to be associated with the inhibition of prostaglandin synthesis. Ketoprofen is used for pain relief and to treat rheumatoid arthritis, osteoarthritis, dysmenorrhea, and other muscle and joint conditions.1 It belongs to Class II of the Biopharmaceutical Classification System (BCS). Ketoprophen is poorly soluble in water, i.e. 0.5µg mL-1at 37ºC.
Poorly water-soluble drugs are associated with slow drug gastrointestinal absorption leading eventually to inadequate bioavailability.2) Various solubility enhancement techniques are considered, such as particle size reduction, pH adjustment, co-solvency, complexations and solid dispersions, being the latter one of the most commonly used.3 In these solid dispersions, the poorly soluble drug is dispersed in diverse types of carriers, ranging from water-soluble to amphiphilic to lipid-soluble ones.2,3
One of the main reasons for the increased dissolution rate of the drug is that when amorphous solid dispersion is exposed to aqueous media such as distilled water or gastric fluid, the polymer carrier will be dissolved or dispersed. Then, the active principle ingredient (API)can be released as very fine, colloidal particles from the solid dispersion dosage form. The increase in surface area of the API as a consequence of its dispersion is claimed to be one of the main reasons of its increased dissolution rate, hence increasing its bioavailability.4
Carriers used to prepare ketoprofen solid dispersions include Gelucire 44/14, Poly(N-vinyl-2-pyrrolidone) (PVP) K30, D-mannitol, poly(vinyl alcohol) (PVA), mixtures of some of them, and chitosan.4,5 It has been reported that solid dispersions prepared using the solvent evaporation technique with chitosan increased the dissolution of ketoprofen. The effect being dependent on the drug/polymer weight ratio and on the molecular weight of chitosan.6
Chitosan (CS) is a linear cationic polysaccharide composed of β(1→4) linked N-acetyl-D-glucosamine and D-glucosamine units. It is obtained by extensive deacetylation of chitin, a natural polysaccharide widely distributed in nature, where it is found in arthropods, insects, arachnids, fungi and algae, among others.7 CS possesses excellent biological properties, such as biocompatibility, biodegradability and low toxicity. It also exhibits other interesting properties such as mucoadhesivity, antimicrobial activity and wound healing capacity.8 The main drawback of CS for many applications is its insolubility in water. To overcome this problem, various water-soluble CS derivatives have been prepared.
Carboxymethyl chitosan (CMCS) is a water-soluble CS derivative that conserves its biological properties. The two general procedures used to prepare CMCS are the reductive alkylation of the -NH2 group with glyoxylic acid, followed by reduction of the Schiff base with NaBH4 or NaCNBH3, and the direct alkylation reaction with monochloroacetic acid and isopropanol.9 The former produces N-CMCS with some proportion of the di-substituted derivative, N,N-CMCS. On the other hand, direct alkylation causes substitution at the -NH2 group, and at the C3 and C6 OH groups. Substitution usually is in the order OH-6 > OH-3 > NH2. Depending on the reaction conditions (pH and temperature) one can obtain O-carboxymethyl chitosan (O-CMCS), N,O-carboxymethyl chitosan (N,O-CMCS) or N-carboxymethylchitosan (N-CMCS). Therefore, the term carboxymethyl chitosan refers to a family of water-soluble chitosan derivatives differing in composition. The composition and the degree of substitution of a CMCS sample influence its physical chemical and biological properties, and should be specified when proposed for a particular application.
Like CS, CMCS has been used in tissueengineering applications as well as in the design of formulations for the delivery of anti-inflammatory drugs, anticancer drugs and for the delivery of proteins, peptides and vaccines, as well as in gene therapy applications.10) A controlled release system based on a pH-sensitive hydrogel composed of N,O-CMCS and sodium alginate (ALG) blended with the naturally occurring cross-linking agent genipinwas developed for protein drug delivery.11 Such combination of genipin with ALG and N,O-CMCS results in an interpenetrating polymer network (IPN) generating a mechanically strengthened and reinforced hydrogel system. The hydrogel exhibited a pH-sensitive drug release pattern and resulted a promising polymeric carrier for site-specific bioactive protein delivery in the intestine. In a further study, hydrogel microcapsules were obtained by dropping aqueous ALG-CMCS mixture into a Ca2+ solution. These microcapsules were evaluated as a pH-sensitive system for delivery of BSA and as in the previous study, it resulted adequate for protein delivery to different regions of the gastrointestinal tract.12 More recently, composite CMCS-ALG gel beads were prepared by dual ionic gelation: the ionic gelation of sodium alginate with Ca2+ and the one between CMCS and β-sodium glycerophosphate (β-GP). The composite gel also resulted appropriate for the delivery of proteins in the intestine and successfully resolved the burst release observed in the alginate-Ca2+ gel system in acidic solution.13
The aim of the present study is to prepare adelivery system for ketoprofen based on Ca2+ cross-linked CMCS-ALG microparticles prepared by spray drying. To this end, CMCS was previously obtained by direct alkylation of chitosan and characterized by various techniques. Ketoprofen loaded Ca-CMCS-ALG microparticles were produced by spray drying andfurther cross-linking with a CaCl2 aqueous solution. Release experiments were performed at pH 1.2 and 7.4, to investigate the pH sensitivity of microparticles.
Materials and methods
Materials
Ketoprofen (KETO), alginic acid sodium salt from Macrocystispyrifera (medium viscosity) and phosphate buffered saline tablets were obtained from (Sigma-Aldrich, Mo, USA). Chitosan (Chitopharm S) was purchased from Chitinor AS (Haugesund, Norway), monochloroacetic acid from Merk (Schuchardt, Germany), isopropanol from Riedel-de-Haën (Seezise, Germany) and CaCl2 from Sigma Chemical Co. (Steinheim, Germany). All other reagents were analytical grade.
Chitosan characterization
Chitosan degree of acetylation (DA). The degree of acetylation (DA) was calculated from C/N ratio according to Eq. 1.14
The molecular weight (Mv) was measured by viscometry using the solvent system 0.3 M acetic acid-0.2 M sodium acetate, and was calculated with the Mark-Houwink-Sakurada equation.15
Preparation of carboxymethyl chitosan
CMCS was prepared by a previously described method 16 with minor modifications. Briefly, 5 g chitosan and 6,75 g sodium hydroxide were added into 50 mL of a solvent mixture (10 mL water, 40 mL isopropanol) and left to swell and alkalize for 2 h at 60 ºC. Then, 7,5 g monochloroacetic acid dissolved in 10 mL isopropanol was added drop-wise for 30 min. After continued stirring for 4 h at 60 ºC, 200 mL of 70 vol.% ethyl alcohol was added. The solid was filtered out, rinsed with 90 vol.% ethyl alcohol and dried at 60 ºC.
Purification was carried out as described by Kong.17 First, 2 g of the product were dissolved in 200 mL distilled water and filtrated after stirring for 30 min. Then, 600 mL of 70 vol.% ethyl alcohol was added to the filtrate and the precipitate was washed consecutively with 70, 80 and 90 vol.% ethyl alcohol and dried at 60 ºC. It was labelled as Na-CMCS, the sodium salt of CMCS.
To obtain the acid form of carboxymethyl chitosan, 1g of Na-CMCS was suspended in 100mL of 80 vol.% ethyl alcohol. Then, 10mL of 37 wt.% HCl was added and stirred for 30 min. The solid was filtered off, rinsed successively with 70, 80 and 90 vol.% ethyl alcohol to neutral, and dried at 60 ºC. It was labelled as CMCS.
Preparation of KETO loaded CMCS -ALG-Ca microparticles
Aqueous Na-CMCS solutions and alginate (ALG) solutions were prepared and mixed at different compositions (CMCS-ALG 0:1 = 0 wt.%: 1 wt.%, CMCS-ALG 1:1 = 0,5 wt.%: 0,5 wt.%, CMCS-ALG 1:2 = 0,34 wt.%:0,66 wt.%). To 50 mL of each mixed solution, 50 mg of KETO were added. Then, 2 mL of 70 vol. % ethanol and 2 wt. % NaOH were added with stirring until drug dissolution. The final pH was 13. The resultant solution was spray-dried using a Büchi Mini Spray Dryer B-290 (Flawil, Switzerland) with a standard 0,5 mm nozzle. Spray drying conditions were set as follows: flow rate 32 m3h-1, compressed air flow rate 473 NLh-1 and inlet temperature 160 °C.
To achieve crosslinking, 50 mg of KETO loaded CMCS-ALG microparticles were immersed into 2 mL of 4M CaCl2 for 30 min at 25 ºC with casual agitation. The microparticles were separated by centrifugation in a Hettich Centrifugen micro (Herrenberg, Germany) at 10 000 rpm for 5 min. After separating the supernatant, the CMCS-ALG-Ca microparticles were washed with water and lyophilized.
Elemental composition and degree of acetylation
The elemental composition (C, N, O) was determined using a using an elemental analyzer (LECO CHNS-932, LECO Corporation, Mi, USA
Determination of isoelectric point of CMCS
Isoelectric point was estimated according to the method reported by Kong.17 Na-CMS (250 mg) was dissolved in 250 mL of 0.1M NaCl aqueous solution, and was divided into five parts in equal volumes. Then, their pH was adjusted to 3, 4, 4.5, 5 and 6, respectively, by adding 0,1M HCl. The precipitate was separated by centrifugation at 8000 rpm. Next, the pH of the supernatant was adjusted to 8 by adding 0.1M NaOH, and the absorbance was recorded at 220 nm in a SP-754 UV-Vis spectrophotometer (Shanghai Spectrum Instruments Co., Ltd., China). The pH corresponding to the minimum absorbance was taken as the isoelectric point.
FTIR spectroscopy
FTIR spectra were obtained with KBr discs and recorded in the spectral range from 4000 to 400 cm-1 by using a by using a FT-IR Nicolet Magna 750 spectrometer. Spectra were obtained with a resolution of 2 cm-1 and were averaged over 100 scans.
1 H-NMR spectroscopy
Polymer samples were dissolved in D2O (10 mgmL-1) overnight and placed into 5 mm NMR tubes. Measurements were performed on AMX 500 Bruker spectrometer (Bruker, Ettinglen, Germany). For 1H-NMR experiments, the conditions used were actual pulse repetition time 2, 5 s and number of scans 128. The spectra were acquired at room temperature. Water signal was presaturated.
The 1HNMR spectrum of CMCS was used to determine the degree of carboxymethylation of CS.
X-ray diffraction
X-ray powder diffraction spectra were recorded using a PHILIPS X’PERT MPD diffractometer (Philips, Amsterdam, The Netherlands), using Cu Kα, radiation operated at a voltage of 40 kV. The samples were analysed in 2-theta angle range 5-50. The process parameters were set at as scan step size 0,040°, scan step time of 1 s, room temperature.
Scanning electron microscopy
The shape and surface of the microcapsules were observed under scanning electron microscopy(SEM) with a JEOL JSM-6400 microscope (Jeol, Tokyo, Japan) at 10 kV accelerating voltage. The samples were previously sputter coated with Au/Pd using a vacuum evaporator (Balzers SDC 004 Sputter coater, Oerlikon Corporate Pfaffikon, Altendorf, Switzerland).
Spray drying production yield
The efficiency of the spray drying process was gravimetrically determined using equation (2)
where theoretical weight is the sum of the CMCS, ALG and KETO weights in the feed.
Encapsulation efficiency
To determine the encapsulation efficiency (EE) a known amount of microcapsules were crushed in a glass mortar and digested in 0,1 N HCl at 37 ºC under strong mechanical stirring (1600 rpm) during 24 h. The samples were centrifuged at 20,000 rpm (Hettich Centrifugen micro, Herrenberg, Germany) for 3 min and the absorbance of the supernatant was determined at 254 nm. Preliminary studies showed that no polymer interference occurs at this wavelength. A calibration curve was prepared by dissolving KETO in 0, 1 N HCL in appropriate concentrations (r = 0,995). EE was determined using the equation (3).
Ketoprofen release experiments
A known amount of microparticles was introduced in a dialysis membrane (Cut-off 12kDa, Sigma-Aldrich, Mo, USA n) along with 1 mL of release media. The membranes were put into closed flasks containing 250 mL of release buffer. Release was carried out at 37 ºC and under orbital shaking (100 rpm).
At pH 7,4, samples were withdrawn at different times and fresh media was added to maintain constant volume. Due to the low solubility of KETO at acid pH, each time the whole volume was removed and was replaced with the same volume of fresh media. Sink conditions were kept during all the experiment. The amount of KETO was determined spectrophotometrically as described in the previous section. Calibration curves were prepared by dissolving KETO in the release buffer (HCl 0,1N or phosphate buffered saline pH 7,4, Sigma-Aldrich, Mo, USA).
Zeta potential determination
Zeta potential of microparticles in 1 mMNaClwas measured using a Nano-Zetasizer system (Malevern Instruments, Herrenberg, Germany). Every determination was carried out in three serial measurements. Electrophoretic mobility and Zeta-potential were obtained for each batch of microparticles.
Results and discussion
Characterization of carboxymethylchitosan
The CMCS prepared by the present method was soluble in water at neutral pH. Owing to its amphiprotic character, it was also soluble at acid and basic pH, with isoelectric point at pH 5.
The molecular weight of the starting chitosan measured by viscometry was 1,01 × 105 g/mol. The elemental compositions of CS and CMCS are reported in table 1. From these data, a DA of 12 % was estimated for CS using equation (1). It can be appreciated the expected relative increase experienced by the C/N ratio for CMCS resulting from the carboxymethylation reaction.
Table 1 Elemental composition in terms of C, H and N for chitosan and carboxymethyl chitosan.
Sample | C (%) | H (%) | N (%) | C/N | DA |
CS | 39,6 ± 0,4 | 6,9 ± 0,3 | 7,4 ± 0,3 | 5,35 | 0,12 |
CMCS | 35,9 ± 0,5 | 5,7 ± 0,4 | 5,0 ± 0,5 | 7,2 ± 0,8 |
In the figure 1 shows the infrared spectra of CS, CMCS and Na-CMCS. The infrared spectrum of CS (figure 1a) displays the distinctive absorption bands at 2942-2784 cm-1 (aliphatic C-H stretching band), 1650 cm-1 (amide I) and 1598 cm-1 (NH2 bending) and 1321 cm-1 (amide III). The absorption bands at 1154 cm-1 (anti-symmetric stretching of the C-O-C bridge), 1082 and 1032 cm-1 (skeletal vibrations involving C-O stretching) are characteristic of its saccharide structure.2 The IR spectrum of CMCS shows the distinctive absorption band of the -COOH group at 1740 cm-1. This spectrum differs substantially from the IR spectrum of CS in the 1450-1300 cm-1 region as results of the overlapping of the band at 1414-1401 cm-1, corresponding to the CH2COOH group, with the amide III band of chitosan. This indicates that carboxymethylation has occurred on both the amino and hydroxyl groups of chitosan. In the IR spectrum of the sodium salt of CMCS, NaCMCS, the characteristic band of the carboxylic group shifts to 1596 cm-1, and the absorption bands at 1154-1029 cm-1 (C-O stretching) also increase, as expected from the transformation of -COOH group into COONa.9
In the 1HNMR spectrum of CMCS (figure 2), a and b are the resonances of H1 D (H1 of the deacetylated unit) and H1 A (H1 of the acetylated unit), respectively, d is the sum of the resonances of H 2A and H 3-6 (6 protons), f is the resonance signal of the protons from N-CH2COOD group and g is the resonance of H 2D. The absence of a resonance signal c at 4,5 ppm, corresponding to one proton from de H3’ (the symbol refers to protons of the carboxymetylated unit) indicates that there was no substitution of caboxymethyl groups at O-3. The degrees of substitution at positions O-6 and N-2 were obtained from the NMR spectrum using the method of Hjerde et al.18
The formulas used for the calculation were:
Where I i is the intensity of the signal labelledi and f 2, f 3 and f 6 are the fractions of carboxymethylation at positions N-2, O-3 and O-6, respectively. F is the total fraction of carboxymetylation.16) The degrees of substitution obtained were f 2 = 0,21, f 3 = 0 and f 6 = 0,88, which gives a total degree of carboxymethylation of 1,09.
Calculation of the C/N ratio for the degree of substitution of 1,09 gives a value of 7,58, which is in good agreement with the C/N ratio of 7,2 ± 0,8 obtained by elemental analysis (table 1). For this composition, that the proportion of free amino groups in CMCS, which were almost 88% in chitosan (DA = 12 %), have now decreased to approximately 70 %.
The X-ray diffraction of pattern of CS in figure 3 shows two intense peaks located at 2θ = 10,20º and 20º, respectively. This corresponds to the characteristic spectrum of the hydrated chitosan described by Clark and Smith.19 In contrast, in the diffraction pattern of CMCS, the peak at 2θ = 10, 20º is absent and only a broad diffraction peak with a maximum located at approximately 2θ = 20,11º is observed, indicating a severe decrease in crystallinity resulting from the carboxymetylation reaction. This was previously reported and it has been attributed to the decrease in the number of hydrogen bond formation between CMCS molecules as compared to CS. This is the direct consequence of the substitution of the hydrogen atoms of the hydroxyl and amino groups of CS by the carboxymethyl groups. Consequently, the ordered arrangement of CMCS chains is decreased with respect to CS reducing its crystallinity.20,21
Ketoprofen-loaded CMCS-ALG microparticles
Microparticles were readily obtained by spray drying. The yield of microparticles prepared at the three compositions was around 55-60 wt.%. This low yield is characteristic of this technique when processing small volumes of solutions, due to the loss of material at the walls of the equipment. When greater volumes of solutions are used, the relative weigh of this loss becomes less important resulting in higher yields.
Scanning electron micrographs of KETO-loaded CMCS-ALG microparticles obtained (figure 4) showed that they had almost spherical shape and were highly polydisperse, with particle sizes between 1 and 10 μm. No drug crystals were observed on the microparticles surfaces. No relevant differences were observed regarding size and polydispersity from one formulation to another while some morphological differences were observed in formulation CMCS-ALG 1:2 since the spheres collapsed during drying.
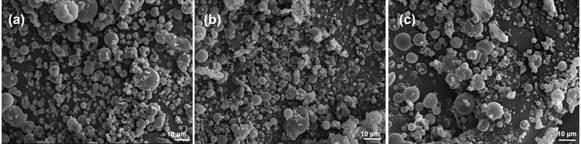
Fig. 4 Scanning electron micrographs of KETO-loaded microparticles prepared by spray drying. (a) CMCS-ALG 1:2; (b) CMCS-ALG 1:1 and (c) CMCS-ALG 0:1.
The zeta potential of the microparticles was determined and results are shown in table 2. In all cases, an overall net negative surface due to the presence of negatives charges from ALG and CMCS was observed. This high negative surface charge allows a good dispersion in aqueous media due to the electrostatic repulsion between particles.
The encapsulation yield was around 50 % for ALG microparticles (CMCS-ALG 0:1) and 60-65 % for microparticlesCMCS-ALG1:1 and CMCS-ALG1:2.
Table 2 Zeta potential values of microparticles
Sample | Zeta potential, mV |
CMCS-ALG 0:1 | -44 ± 4 |
CMCS-ALG 1:1 | -51 ± 5 |
CMCS-ALG 1:2 | -63 ± 5 |
Figure 5 shows the IR spectra of sodium alginate and the CMCS-ALG microparticles. The IR spectrum of ALG presents the characteristic bands around 1030 cm-1 (C-O-C stretching) attributed to its saccharide structure. In addition, the bands at 1617 and 1417 cm-1 are assigned to asymmetric and symmetric stretching bands of carboxylate salt groups.22 These bands are also present in the spectra of CMCS-ALG microparticles which are very similar to that of ALG. This is to be expected from the similarity of the spectra of both polysaccharides. However, two main differences are observed. The first one is the shift of the band at 1303 cm-1 present in ALG and CMCS-ALG 0:1 microparticles to 1327 cm-1in the spectra of CMCS-ALG 1:2 and CMCS-ALG 1:1 microparticles due to the contribution of the band at 1324 cm-1 in CMCS (Figure 1). The second one is that the relative intensity of the band at 1088 cm-1 with respect to the band at 1030 cm-1 is grater in the spectra of microparticles containing CMCS than in ALG and CMCS-ALG 0:1. It is worth mentioning that the characteristic absorption bands of KETO are not detected in the spectra of microparticles. This must be due to the facts that KETO concentration in microparticles is only around 5 - 6,5 % and the characteristic absorption bands of KETO at 1655 cm-1(C=O stretching of ketone), 1595 cm-1 (aromatic C=C bond), 1440 cm-1(asymmetrical C-H deformation of CH3) 5) are masked by the absorption bands of ALG and CMCS in these zones. Moreover, the distinctive band of KETO at 1693 cm-1(C=O stretching of acid) is absent in the spectra of microparticles, because in them the drug is in the carboxylate form. The asymmetric and symmetric stretching bands of the carboxylate groups of the carboxylate form of KETO appear at 1567and 1394 cm-1, respectively 23, which are in the same region than the corresponding ones of ALGand CMCS carboxylate groups.
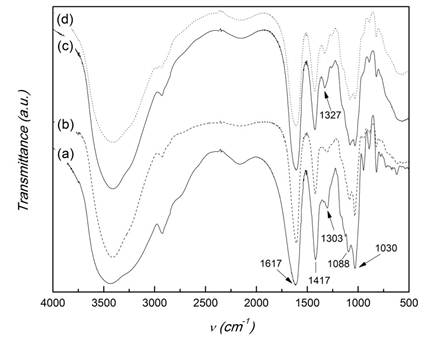
Fig. 5 FTIR spectra of sodium alginate and CMCS-ALG microparticles. (a) ALG; (b) CMCS-Alg 0:1; (c) CMCS-Alg 1:2; (d) CMCS-Alg 1:1.
The diffraction patterns of ALG and microparticles are displayed in figure 6. The peaks of ALG at 2θ values 13,5º, 22º and 37º are due to the reflection of their (110) plane from polyguluronate unit, the (200) plane from polymannuronate and the other one from the amorphous halo.24) KETO is a crystalline material with characteristic diffraction peaksappearing at 2θ values 6,33, 13,16, 14,42,16,22, 17,30, 18,42, 19,15, 20,04, 22,91, 23,95, 27,23, 27,73,29,49º, etc.5) The absence of these diffraction peaks in the X-ray diffractograms of microparticles indicate that KETO in microparticles is in an amorphous state.
In vitro drug release experiments
A pharmaceutical form intended for the oral route goes to the stomach, and after residing there for a definite time-period (15 min to 4 hours), it passes first to the small intestine, and finally to the colon. In this process, the formulation is exposed to a sharp pH change in the range 1 - 2 (gastric fluid) to 7 -8 (intestinal fluid). Therefore, we studied the release of KETO in a simulated gastric fluid at pH 1.2 and in a simulated intestinal fluid at pH 7.4.
The in vitro release profiles of KETO loaded cross-linked with CaCl2microparticlesat pH 1,2 and 7,4 in a short-term experiment is shown in figure 7. In both media, a controlled release was observed with an initial burst for the three compositions tested. CMCS-ALG1:1 and CMCS-ALG1:2 microparticles showed lower KETO release than CMCS-ALG 0:1microparticles. KETO release from CMCS-ALG 0:1 microparticles was pH dependent while KETO release from the other two CMCS-ALG microparticles was almost independent onpH.
In a previous work, Sorasuchart et al.25 found that the release kinetics of ionizable drugs from a nonpH-dependent polymer (ethyl cellulose) was pH-dependent. In their study, KETO was released faster in basic medium than in acidic one owing to its more ionized state in basic medium.
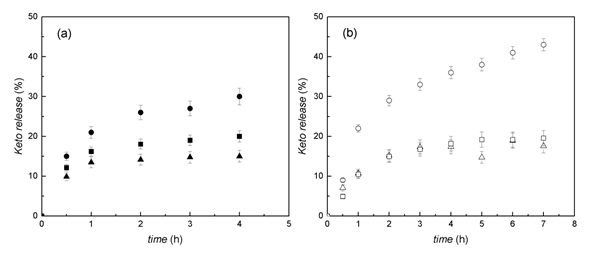
Fig. 7 KETO release at 37 ºC and 100 rpm. (a) Simulated gastric media (HCl 0.1 N,pH= 1.2) and (b) simulated intestinal fluid (saline phosphate buffer, pH= 7.4). Circles, CMCS-ALG 0:1 microparticles; squares, CMCS-ALG 1:1 microparticles; triangles, CMCS-ALG 1:2 microparticles. Each experiment was carried out in triplicate and data are presented as mean ± SD.
The release pattern of microparticles in a longer-term experiment (up to 20 days) at pH 7,4 is shown in figure 8. In this longer-term experiment, a prolonged KETO release was observed. CMCS-ALG 0:1microparticles released almost 75 % of KETO whereas the other two CMCS-ALG formulations released about 40 % of the drug, with little difference between them. In all cases release increased almost linearly with time without reaching a plateau.
Similar prolonged release was reported by Prabaharan et. al26 from KETO loaded carboxymethyl chitosan-graft-phosphatidylethanolamine (CMCS-g-PEA) beads. In their report KETO release from CMCS-g-PEA beads at pH 7.4 was only about 58 % at 20 hours, although it reached almost 9 0% after 50 hours.
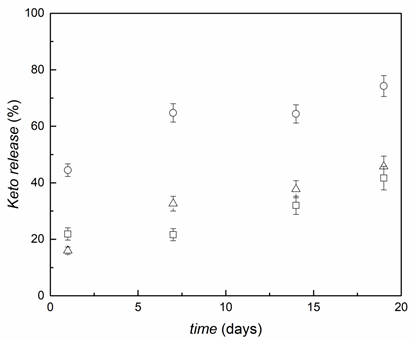
Fig. 8 Long-term KETO release in simulated intestinal fluid (saline phosphate buffer, pH=7.4, 37 ºC, 100 rpm). Circles, CMCS-ALG 0:1 microparticles, squares, CMCS-ALG 1:1 microparticles; triangles, CMCS-ALG 1:2 microparticles. Each experiment was carried out in triplicate and data are presented as mean ± SD.
The release of a drug from a polymer matrix depends on multiple factors, such as matrix porosity, hydration and erosion, polymer chain relaxation, ionic and hydrogen bonding interactions andhydrophobic interactions. When the polymer components of the matrix carry ionic or ionizablegroups, polymer-polymer and polymer-drug ionic interactions acquire particular relevance and the pH of the media is an important parameter affecting the ionization degree, as reflected in Eqs. (8) and (9).25
For weak acids
For weak bases
KETO (weak acid, pKa4.8) is only slightly soluble in water (51 mg L-1 at 22 °C).According to Eq. (8), the ratio of the ionized to the nonionized form of KETO at pH 1.2 is 3.98 ×10-4 while at pH 7.4 is 398. Therefore, thesolubility of the ionized drug at pH 7.4 is greater than at acid pH.
The pKaof ALG is 3.5 and the reported pKa of the -NH2 groups in CMCS is 8.0.27 This implies that at pH 1.2 almost 100 % of the -COOH groups in ALG are nonionized, while all the amino groups in CMCS are protonated. On the other hand, at pH 7.4 the carboxylic groups of ALG are as COO- and 80 % of the -NH2 groups of CMCS are still protonated and can interact electrostatically with ALG and KETO.
It has been reported that calcium ions react extremely fast with alginate occupying all available binding sites on the alginate molecule rendering a gel state.28 The swelling of this calcium-alginate matrix depends on the pH of the media.26 At acid pH (below alginate pKa) swelling is very limited. On the contrary, at pH 7.4, alginate swells due to the exchange of the cross-linking calcium ions with non-gelling Na+ or K+ ions (components in phosphate saline buffer). Moreover, atpH7.4 most of the carboxylate groups are deprotonated and the repulsive electrostatic forces lead to a more extended polymer conformation. Owing to this effect of the pH on the polymeric matrix, it has been found that KETO release from alginate microcapsules is larger in basic media than in acidic one.29
Our spray-dried alginate microparticles cross-linked with CaCl2 showed a similar behavior. That is, larger release in PBS is observed due to highest swelling of alginate in this media and the electrostatic repulsion with the negatively charged KETO molecules. The presence of CMCS altered this pattern because similar release was observed at pH 7.4 and pH 1.2, no matter the assayed formulation (CMCS-ALG 1:1 or CMCS-ALG 1:2). This occurs because the structure of CMCS-ALG microparticles is less affected by calcium exchange in PBS media owing to the electrostatic interaction between the carboxylic groups of ALG and the protonated -NH2 groups of CMCS, which at pH 7.4 are still about 80 %. This effect increases with increasing the CMCS proportion in the microparticle composition, so that KETO release decreases in the order CMCS-ALG0:1>CMCS-ALG 1:2> CMCS-ALG 1:1.
Regarding the percentage of drug released, it appears that the calcium alginate microparticles result more appropriate for the colonic release of KETO. However, the CMCS-ALG 1:1 and CMCS-ALG 1:2 ketoprofen loaded microparticles can be an appropriate system for other applications. For instance, in designing a prolonged release of KETO by administering the drug by the parenteral route.
Conclusions
Ketoprofen was successfully encapsulated in carboxymethyl chitosan-alginate microparticles by spray drying. The encapsulation efficiency was between 50 and 65 %, depending on the microparticles’ composition. X-ray diffractograms showed that the drug in microparticles was in the amorphous state, which favours its dissolution. Release of ketopropfen from CaCl2 cross-linked microparticles was performed at pH 1.2 and pH 7.4. Drug release was pH and composition dependent and higher release was obtained at pH 7.4. At this pH CMCS-ALG 0:1microparticles released almost 75 % of KETO after 20 hours, whereas the other formulations(CMCS-ALG 1:1 and CMCS-ALG 1:2) released only about 40 %. In all cases, release profile was almost linear without reaching a plateau. It is concluded that CaCl2 cross-linked CMCS-ALG microparticles are a promising vehiclefor designing formulations for the prolonged release of ketoprofen