INTRODUCTION
The fertility level of dry acid lateritic soil is very low. The soil is naturally deficient in phosphorus, nitrogen, and other essential nutrients like calcium, magnesium, etc. In this type of soil, nutrients remain unavailable to the plant, resulting in unfavourable conditions for plant growth (Koley, 2000). In conventional agricultural practices, uses of overdose agrochemicals day by day increasing of low fertility and toxicity in the soil in agricultural fields and pesticide-resistant pests throughout India. As a result of these real problems, adverse effects on health are being reflected (Mittal et al., 2013; Rahman and Debnath, 2015). Therefore, in modern agriculture strategy, the huge demand for producing organically toxic-free vegetables is increasing, which also cost-effective and environment-friendly (Madhusudhan, 2016; Pandey and Singh, 2012).
Mycorrhiza is the mutualistic root-fungus relationship between non-pathogenic soil fungi and the plant roots (Frank, 1885; van der Heijden et al., 2015). Arbuscular mycorrhiza (AM), belonging to the phylum Glomeromycota (Redecker et al., 2000; Kapoor et al., 2008), are the most common symbiotic association of mycorrhiza found in 90% of land plants (Schüer et al., 2001; Brundrett, 2002; Smith and Read, 2008). AM colonizes in the cortical tissue of roots during active plant growth (Kirk et al., 2001) and procures nutrient form beyond the nutrients depletion zone of roots (Li et al., 1991). Organic acids and phosphatases produced by AM help to release of available phosphorous from unavailable complexes (Bagyaraj, 1984; Entry et al., 2002; Fomina et al., 2005). They also increase nitrogen and carbon content in soil (Almas et al., 2004). The fine extra-radical hyphae of arbuscular mycorrhizal fungi (AMF) involve in the nutrient process and uptake phosphorous (Bowen, 1973; Bucher, 2007), provide nitrogen (Madder et al., 2000; Chalot et al., 2006; Nuccio et al., 2013) by absorbing ammonium, nitrate and amino acids (Hodge et al., 2001), and deliver other plant micronutrients like copper, zinc, etc. (Smith and Read, 1997), and in exchange, withdraw organic sugar from plant roots (Kottke, 2002).
AM hyphae can absorb water from lower water potential than the plant roots (Bethlenfalvay et al., 1988; Püschel et al., 2020) and form a mycelial mat which promotes retaining soil moisture. AM symbiosis is effective to overcome low pH in acidic soil which restrict plant growth (Clark, 1997; Muthukumar et al., 2014). The increasing water and nutrient uptake ability of AMF in low fertile and dry condition (Smith and Read, 1997; Aúge, 2001) provide improved plant growth in less-nutrient soil, such as acid lateritic soil (Weber et al., 1992; Brundrett, 2009). They also protect plants from pathogenic fungi and nematode disease (Bagyaraj, 1984). Nowadays, arbuscular mycorrhizal inoculation to the crops has become an appreciable alternative that increases the growth and yield (Mishra and Verma, 1982; Johansen et al., 1993; Ghosh and Verma, 2006; Samanta and Verma, 2006; Sengupta et al., 2006; Robinson et al., 2016; Chukwuka et al., 2017).
Acaulospora is the AM fungal genus belonging to the family Acaulosporaceae, has been distributed in more than 30 species (Simanungkalit, 2006). Acaulospora sp. are a common dominant group of arbuscular mycorrhiza at the lateritic ecosystem (Ghosh and Verma, 2011). A special feature, having the sporiferous saccule with the saccule subtending hypha present in Acaulospora (INVAM, 2018).
The plant-growth-promoting rhizobacteria (PGPR), present in plant mycorrhizosphere, promote the growth of the plant (Andrade, 2004; Giri et al., 2005). Azotobacter and phosphate solubilizing bacteria are the important plant growth promoters (PGP) and act as mycorrhiza helper, having an important role in the growth of hyphae from germinating AM spores, colonization of plant roots by AM fungi and growth of external AM hyphae and dehydrogenase activity of the AM fungus (Burla et al., 1996). Azotobacter is free-living soil bacteria, fix free nitrogen directly from the environment. Inoculation of Azotobacter sp. to the plant has showed improved beneficial effects in seed germination, shoot, root biomass, and yield (Kumar et al., 2000; Kumar et al., 2009; Malik et al., 2009; Reddy et al., 2003). Phosphate solubilizing bacteria (PSB) can release bound phosphate to the available P form (Bhattacharyya and Jain, 2000; Ponmurugan and Gopi, 2006). Many research works related to plant growth promotion by the application of phosphate solubilizers have been reported earlier (Khiari and Parent, 2002; Saxena and Sharma, 2003; Yazdani et al., 2009).
Chilli (Capsicum frutescens L.) is the part of the Solanaceae family, is a very essential ingredient in our daily vegetables. It is rich in volatile oils, fatty oils, capsaicinoids, carotenoids, vitamins, proteins, fibers, and minerals and acts as a natural bactericidal, having medicinal uses to treat muscle pain, cough, asthma, sore throat, etc. (Bosland and Votava, 2000). As it is an economically important crop, the average yield of chilli in India is lower as compared to other developed countries (APEDA, 2019; FAOSTAT, 2017). Cropping in unused barren land containing infertile soil such as lateritic soil may become a suitable solution.
The present research was conducted to determine the primary impact of singly and combined application of native bio-inoculants, the AM, Acaulospora, and the PGPR, Azotobacter and Pseudomonas sp. (PSB) on growth and yield of chilli (Capsicum frutescens L.), growing in acid lateritic soil.
MATERIALS AND METHODS
Plant material
Certified seed of chilli (Capsicum frutescens L.) were collected from District Agricultural Head office, Paschim Medinipur, West Bengal, India. Healthy seeds were selected manually and surface disinfected by immersion in an aqueous solution of 0.1% (w/v) mercuric chloride (HgCl2) for 3-4 min followed by washing three times with autoclaved deionized water under aseptic conditions in a laminar flow chamber.
Experimental conditions
The experiment was conducted with chilli (C. frutescens) planted in sterilized lateritic soil (Sylvia, 1994). It was done in 22.30° N Latitude and 87.20° E Longitude in Midnapore subdivision of West Bengal in the pre-winter season. The primary soil characteristics were tested according to Jackson (1973), having pH 5.61, electrical conductivity (EC) of 0.18 m mohs/cm2, moisture content of 2.7%, organic carbon (OC) of 0.63 g kg-1, total nitrogen (N) of 0.04% and phosphate (P) of 0.03%.
Isolation of the AM propagules
Separation and isolation of indigenous AM propagules from the native ecosystem was done by following the wet sieving and decanting technique (Gerdermann and Nicolson, 1963). Spores of single species were separated morphologically using a LABOMED CSM2 microscope, and surface disinfected by 200 μg ml-1 streptomycin and 2% Chloramine-T solution (w/v) (Mosse, 1973).
The pure culture of each inoculum was cultured in sterilized sand and soil mixture. The soil: sand (1:1, v/v) mixture was sterilized by oven drying at 85 °C for 8 h with a gap of 48 h and again dried for 8 h (Sylvia, 1994). Pure cultures were done in funnels and mass cultures in surface-disinfected earthen pots, grown in an automated growth chamber with sorghum (Sorghum vulgare L.) plants. The mass cultures were maintained up to 90 days in the growth chamber at 26±2 °C under a photoperiod of 16 h light and 8 h dark, an irradiance provided by 110 W fluorescent lamps (Philips, India).
The successful mass culture of the indigenous AM inocula was identified morphologically by following Schenck and Perez (1990) and the INVAM web photo guide (INVAM, 2018) and confirmed as Acaulospora sp.
Bacterial cultures
Azotobacter was isolated from lateritic rhizospheric soil in Ashbey mannitol agar media for free-living nitrogen-fixing bacteria (Pelczar et al., 1957; Subba, 1977). Pikovskaya media for native phosphate solubilizing bacteria (Pikovskaya, 1948; Sundara and Sinha, 1963; Subba, 1977) were used. Azotobacter sp. and Pseudomonas sp. (PSB) were confirmed according to Bergey manual (Holt and Krieg, 1984; Krieg et al., 1994).
Seedling inoculation
Surface disinfected seeds of chilli were germinated in aseptic conditions and seeded with a 10 cm gap from each other in a seedling tray within a growing chamber under the environment at temperature 25-30 °C, humidity 65-70% and 15-16 h of lighting. Sterilized soil and sand mixture in 2:1 (v/v) ratio was used as growing substrate.
After germination, the healthy chilli seedlings were transferred to polythene bags (18 x 24 cm) filled with 3 kg sterilized soil (one seedling per pot) to grow for 90 days in the greenhouse. AM inoculation was done by soil-based inoculum obtained from mass culture (20 spores /g, 25 g /pot), applied under 4 cm from the soil surface. 10 ml of the bacterial cultures contained about 108 CFU ml-1 liquid medium were applied per pot.
The experiment was designed in a randomized block with eight treatments and three replicates. The treatments of this experiment consisted with T0 = No inoculation (Control), T1 = Azotobacter (AZO), T2 = Phosphate solubilizing bacteria (PSB), T3 = Acaulospora sp. (Ac), T4 = Azotobacter + PSB (AZO+PSB), T5 = Acaulospora sp. + Azotobacter (Ac+AZO), T6 = Acaulospora sp. + PSB (Ac+PSB), T7 = Acaulospora sp. + Azotobacter + PSB (Ac+AZO+PSB).
Plant parameters were measured at 30, 60 and 90 days after plantation (dap) in three replicates per treatment in terms of shoot height (cm), leaf number per plant, leaf area (cm2), the total number of flower and fruit appeared per plant, and total fresh weight (g) and dry mass (g) of fruit yield per plant. The fresh yield was calculated by summing the total fresh weight of fruits obtained. The dry mass was measured by drying the fruits in a hot air oven at 65 °C for 48 hours until a constant weight was obtained.
Rhizospheric soil samples were tested for the AM spore population by wet sieving and decanting technique (Gerdermann and Nicolson, 1963). Plant root samples were treated with 10% KOH and stained with tryphan blue (Phillips and Hayman, 1970) to study the colonization percentage by the equation 1:
Statistical analysis
Statistical analysis of data was done by comparison of means using the least significant difference (LSD) at p < 0.05 probability, after the preforming analysis of variance (ANOVA) using IBM SPSS 20 and MS Excel 2013. Pearson correlation coefficient between variables was analyzed at p < 0.05 and p < 0.01 level of significance.
RESULTS AND DISCUSSION
The inoculation of chilli seedlings with an indigenous AM and PGPR combination increased the growth since the early stage, with prominent effect of AM.
At 30 dap, the treatment T7 having Acaulospora sp. + Azotobacter + PSB (Ac+AZO+PSB) showed height, leaf number, leaf area and root collar diameter slightly superior. Nevertheless, after 60 days of culture, the plants in treatment Ac+AZO+PSB (T7) were 23.66% higher than the control, followed by Ac+AZO (21.3%), Ac+PSB (15.5%) and Ac (10.6%). The same pattern was observed in the total leaf number and root collar diameter. Leaf area was in Ac+AZO+PSB 14.3%, in Ac+AZO 11.1% and in Ac 10.0% higher than the control.
The first appearance of flowering have few days of difference among the treatments. It was observed on 26th day after plantation in AZO+PSB and Ac+AZO+PSB, at 28th day in Ac+AZO and at 29th day in AZO, Ac, and Ac+PSB. On the other hands, first fruiting was observed at 33rd dap in AZO+PSB followed by Ac+AZO+PSB and Ac+AZO at the 36th day; and Ac and Ac+PSB at 41st day.
After 90 dap, in treatment with the combination of AM and PGPR (Ac+AZO+PSB, T7) all the parameters reached the maximum, without significantly differences with Ac+AZO (T5) in plant height, leaf number and root collar diameter. As well as T7 treatment was similar to T6 (Ac+PBS) in root collar diameter (Table 1). The shoot height in Ac+AZO+PSB was 31.64% superior to control and the leaf number 37.8%. The total fruit number was found maximum in Ac+AZO+PSB, 127.5% better than control and significantly higher (p<0.05) all the rest. A similar result was observed in the total fresh and dry mass of fruits yield per plant (Figure 1).
Table 1. Effect AM and PGPR on chilli (Capsicum frutescens L.) plants parameters after 90 days after plantation.
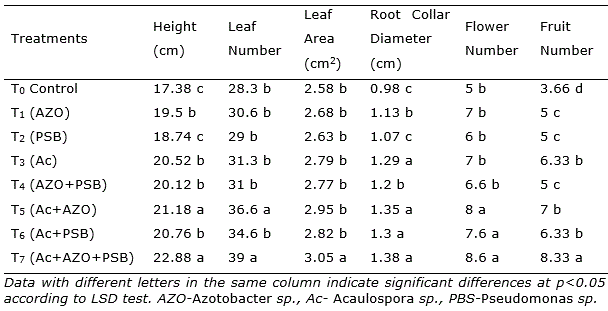
In single inoculation, the effect of AM was superior than Azotobacter and PSB in root collar diameter and fruit number. In dual inoculation, the combination of AM and Azotobacter was better than AM and PSB in height and leaf number. Plants inoculated with Azotobacter sp. have shown an increase in shoot and yield effects. It have been tested on different crops (Reddy et al., 2003; Singh and Rana, 2005; Kumar et al., 2009; Malik et al., 2009). The dual effect of inoculation of AMF and Azotobacter was found encouraging in earlier research work also (Paul et al., 2011; Dal et al., 2018; Shaimaa and Massoud, 2017). Azotobacter adds only nitrogen in soil and PSB release available phosphate, AM mainly translocate nutrients including micronutrients through the extensive mycelial network (Kayama and Yamanaka, 2014; Rouphael et al., 2015) and also release phosphates by phosphatase (Zhang et al., 2014).
Hence, AM alone is found almost sufficient for nutrition rather than Azotobacter or PSB in single inoculation. In dual treatments, also uptake ability of AM was in advance than the only supply of nutrients, and Azotobacter as a source of N and AM as P substitute worked best. Interaction between the AM, Acaulospora with Azotobacter and PSB, in this triple application may provide the development of plant growth and yield (Nadeem et al., 2014; Hashem et al., 2016). Enhanced supply of N and P in the rhizosphere by PGPR boosts up AM uptake in the triple inoculation resulting establishment of better mycorrhizal activity (Selvakumar et al., 2012; Vafadar et al., 2014; Raklami et al., 2019).
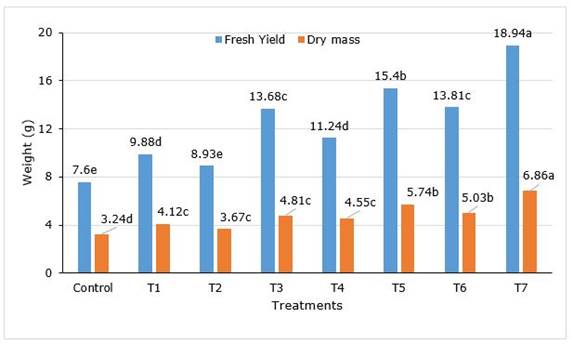
Figure 1. Fresh yield and dry mass of Capsicum frutescens L. inoculated with AM and PGPR. Treatments T1 (AZO), T2 (PSB), T3 (Ac), T4 (AZO+PSB), T5 (Ac+AZO), T6 (Ac+PSB), T7 (Ac+AZO+PSB). AZO-Azotobacter sp., Ac- Acaulospora sp., PBS-Pseudomonas sp.
The mycorrhizal infection and spore population were found maximum in Ac+AZO+PSB (T7) treatment followed by MA combined with PGPR (Ac+AZO, Ac+PSB) (Figure 2). Mycorrhizal colonization was mostly mycelial and arbuscular.
Mycorrhizal colonization with class - III intensity produced in Ac+AZO+PSB and Ac+AZO. In these treatments, colonization percentage were observed higher than the inoculation formed in Ac alone. A strong significant positive correlation (r=0.997, p<0.05 and p<0.01) was observed between AM root colonization and spore density. They were also showed a significant positive correlation (p<0.05 and p<0.01) with all the measured plant parameters, including fresh yield and dry yield. The last one were also positively and significantly correlated (p<0.05 and p<0.01) with plant height.
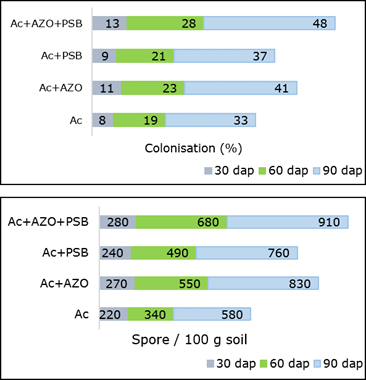
Figure 2. Mycorrhizal colonization and spore population in root and rhizosphre of chilli plant inoculates with AZO-Azotobacter sp., Ac- Acaulospora sp., PBS-Pseudomonas sp.
In all the AM related treatments, arbuscular colonization and spore formation occurred. Formation of arbuscles and high-intensity colonization in the plant roots indicates active symbiosis between Acaulospora and C. frutescens in those treatments. As the natural propagules in lateritic soil are low, the mycorrhizal application to the plant increased the growth and yield (Ghosh and Verma, 2006; Hempel et al., 2013). AM are more active in nutrient-poor dry soils, plants depended more on mycorrhizae for nutrients and moisture in this condition (Brundrett, 2009; Begum et al., 2019). The mycorrhization facilitates an effective hyphal network that spreads throughout the rhizosphere and improves the nutrient absorbing ability of the host plant (Avio et al., 2006; Püschel et al., 2020). Active mycorrhizal colonization in the plants alters some physiological processes and increases water and nutrient uptake which enhances crop growth and yield (Oyetunji et al., 2003; Mardukhi et al., 2011; Douds et al., 2005), which also influenced the chilli plant (Gaur et al., 1998; Vyas and Vyas, 2014).
The test plant, C. frutescens establish showed AM dependency on the dominant indigenous AM genus, Acaulospora, in this lateritic zone (Sieverding, 1991; Ghosh et al., 2008), which shows active adaptation and efficacy in native soil (Oliveira et al., 2005; Samanta and Verma, 2006; Akib et al., 2018). The triple application of AM, Azotobacter, and PSB were found to influence the growth of the crops too (Shwetha and Lakshman, 2013; Lallawmkima et al., 2018), though with other AM species, not indigenous or in lateritic soil.
Enhancement in productivity of chilli through the mycorrhization established in earlier works (Selvakumar and Thamizhiniyan, 2011; Bhuvaneswari et al., 2014). The mycorrhizal inoculation can enhance the yield of chilies significantly and reduced the application of chemical fertilizers by up to 50 percent (Bagyaraj and Sreeramulu, 1982). Application of AM with such mycorrhiza helping rhizobacteria develops a synergistic microbial interaction (Artursson et al., 2006) and maybe a substitute for chemical fertilizer particularly in this dry acidic soil.
CONCLUSIONS
Application of indigenous AM, Acaulospora sp. along with beneficial and native plant growth-promoting rhizobacteria, Azotobacter and PSB facilitate C. frutescens L. better growth and enhanced the yield grown in infertile dry and acid lateritic soil. Such AM and microbial consortium could be benefit other crops also in this soil type and organic agriculture and horticultural practice particularly in other poor nutrient soil and in surely reduced cost in comparison to chemical fertilizers.