Introduction
The wastewater from swine farm is generated from the pig excreta as well as the water used to clean the hog housing sheds. Thus, the wastewater generated in rich in ammonia content from the urine of the hogs, and the manure contributes to the high chemical/biological oxygen demand. Studies on small scale pig farms in china, the leading pork producer in the world, indicated the generation of about 1300 t wastewater per year per pig farm, including manure, urine and washing wastewater. Proper treatment of the waste generated and safe environmental disposal is an integral part of the farms since swine wastewater is highly toxic.1
In Cuba, in 2010 swine production alone accounted for 225 000 t. Many production farms have inefficient systems for residual water treatment thus causing a negative impact on the environment. As a result of their contribution with organic matter, nitrogen, and phosphorus, new means and methods are required for treatment and less aggressive disposal into the environment. 2
To date, conventional physico-chemical and biological treatment methods are widely used for the treatment of wastewater. However, the efficient operation of these systems requires substantial operation and maintenance costs, and the application of novel technologies for the treatment and disposal of sludge/residues.3
Nowadays biological wastewater treatment methods have attracted the attention of workers throughout the world and have helped in developing efficient and low-cost waste treatment systems. These techniques involve the application of anaerobic or aerobic processes to biologically breakdown the organic pollutant present in the industrial wastewater.4
Microalgal biotechnology has gained importance in recent years since its growth requires low-cost substrates and can be effective in obtaining compounds with high added value. In general, microalgae are cultivated in wastewater for carbohydrates, lipids and proteins that are mainly focused on the production of fuel and animal feed. However, microalgae are known to produce high-value bioactive compounds, which can have different pharmaceutical and cosmetic applications. They have the potential to reduce greenhouse gases (GHG), especially carbon dioxide emissions and the ability to assimilate nitrogen and phosphorus from wastewater. 5,6,7
The significant factors that influence the occurrence and accumulation of algal blooms in surface waters are light, temperature, and nutrient concentrations. In favorable conditions, algae and bacteria show symbiotic relationships that remove nutrients from the aquatic environment and remain in ecological balance without supplemental aeration. 8
Kinetic models are an indispensable tool for the study of microalgae productivity. These not only provide a basis for the design of the reactor, but also for the improvement of processes through facilitating the exploration of various environmental and operating conditions.9,10 The kinetic model is utilized to describe dynamic cell growth in a black-box profile because it models relationships on the basis of input (e.g., nutrients, light intensity, and temperature) and output (e.g., biomass and product concentrations) coefficients. It is a valid tool to increase the productivity of target products by optimizing the environmental and culture conditions. (10
In review by 11 26 kinetic models are reported in microalgae, 14 of them consider the intensity of light as the only nutrient, which are often applied on a laboratory scale, but not to a real system with different species.12 Developed a kinetic model that considers the effect of light intensity and dissolved inorganic carbon applying the Verhulst logistic model but the culture conditions differ from that of this study.
In general, the developed and validated models refer to a single species, when in reality the cropping systems can contain several species, mainly in open systems. Therefore, developing a model that takes into account the relationship between microalgae and bacteria better represents the open-pit system.
The objective of this work is to obtain a kinetic model of a mixotrophic culture of Chlorella vulgaris in porcine opencast residuals that considers the effect of solar irradiation.
Materials and methods
Microorganism and general conditions of experimentation
The investigations were carried out at the Solar Energy Research Center (CIES) of the CITMA and the "El Brujo" Pig Combined
The cultivated species is the microalga Chlorella vulgaris, which is conserved in the collection of microalgae of CIES at an average temperature of 25 oC.
The culture experiments were carried out in a 3500 m2 thin-film photobioreactor that is part of an integral waste treatment system located in El Brujo porcine feedlot areas in Santiago de Cuba.13. The experimental data were taken on sunny days from the months of May-July between 07:00 and 16:00 h.
During the night the culture suspension is kept recirculating in a tank of 32,5 m3. The porcine liquid residual was used as the only source of carbon and inorganic nutrients for the cultivation of microalgae. To keep the culture volume constant, residual is added at different time intervals. For them, the % evaporated water due to solar irradiation is taken into account.
The mixed cell concentration was calculated every 15 min from measurements of sample optical density with an UV-Visible spectrophotometer (Spekol 10) at 750 nm wavelength (OD750). At this wavelength it is assumed that there is no absorption by pigments and therefore is directly related to the mixed biomass.
The growth of microalgae and bacteria was calculated twice a day, at 7 and 17 h, by counting cells with a Jenaval binocular optical microscope (in an improved Neubauer chamber of 0,1 mm depth). (14
Taking into account the stoichiometry of the reaction and the mass balances, the concentrations of microalgae and bacteria are calculated every 15 min. 7
The solar radiation values were taken every 15 min, from the CIES actinometric station coupled to a data acquisition system Data logger MP-110 (Japan).6
Mathematical Modeling
The Verhulst logistic kinetic model 15 was used to fit the evolution of biomass concentration. The time evolution of the mixed biomass concentration (X (t)) was calculated by integrating equation (1), as shown in equation (2):
The initial biomass concentration is represented by X0 (in g L-1), the máximum
specific growth rate (
) and the maximum biomass concentration (
). (2).
In this way, equation (3) is established to describe the time evolution of the concentration of microalgae and bacteria).
To obtain the mathematical model of concentration of biomass of microalgae and bacteria as a function of solar irradiation it is necessary first to establish the mathematical models of
y
for microalgae, this is the species that needs solar irradiation for its growth.
Results and discussion
Relationship between microalgae and bacteria
The analysis of the data shows the relationship between microalgae and bacteria through equation 4. As a result of the primary modeling, the kinetic parameters of microalgae and bacteria, maximum concentrations of microalgae and bacteria (,
) and maximum growth rates of microalgae and bacteria (
,
)
are obtained.
Adjusting the linear function lineal Y= A+B*X to the data, we obtain the relation between the maximum concentrations and the growth rates (figure 1 and figure 2).
Equation 5 shows the relationship between the maximum concentrations of microalgae and bacteria and Equation 6 shows the relationship between the maximum growth speed of microalgae and bacteria
Mathematical modeling formulation based on solar irradiation
The behavior of the growth rate and the maximum concentration of microalgae as a function of cumulative solar radiation is described in figure 3. This behavior is described by the second and third order polynomial functions, where Y is the studied response and X is the factor studied.
The values of represent the coefficient of the terms and indicate the curvature. The quadratic model (equation 7) offers a good approximation of the true behavior of the studied system over the dominant factor and allows to obtain the optimal solar irradiation.
From the above data, the equation of maximum growth rate of microalgae as a function of solar irradiation is obtained (equation 8)
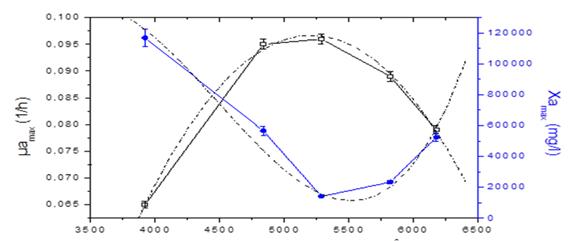
Fig.3 Behavior of the concentration and maximum growth rate of microalgae as a function of cumulative solar irradiation
A correction factor is applied that allows obtaining a better adjustment of the growth curve (equation 9). Deriving the second-degree polynomial function that describes the maximum growth rate, the value of the optimum cumulative solar irradiation is obtained (equations 10 and 11). The optimum accumulative solar irradiation according to the model is 5209 W / m2h.
The maximum concentration of microalgae biomass (Xamax) is determined according to equation 12.
The equation of maximum concentration of microalgae based on solar irradiation is
obtained:
Substituting equations (8) and (13) in (3) and equations (5) and (6), representing the relationship between microalgae and bacteria, by the values of the parameters then we obtain the general model, represented by equations (14), (15) y (16).
Model validation and prediction
To validate the effectiveness of the model, validation is carried out. First, the specific ranges of crop parameters are established:
Initial ratio of biomass:
Substrate function: Fs = 1, 2 ± 0, 5
Initial ratio of biomass: (Xao / Xbo) = 1, 54 ± 0, 06
Temperature: T=29 oC ± 7
Four runs are taken with different behaviors in solar irradiation to evaluate the growth kinetics (figure 4)
Figure 5 shows the growth kinetics of microalgae and bacteria at different solar irradiation accumulated according to the model and the experimental data. It can be observed that the highest concentration of microalgae and bacteria are obtained at 5624 W/m2h and those values above or below this range obtain lower concentrations of biomass. This value is in the range of optimal solar irradiation values (4900-5800) W / m2h obtained in the model.
Figure 6 shows the validation of the model, correlating the experimental values and those obtained by the model. The evaluation is made taking the criterion of the obtained correlation coefficient (R2).
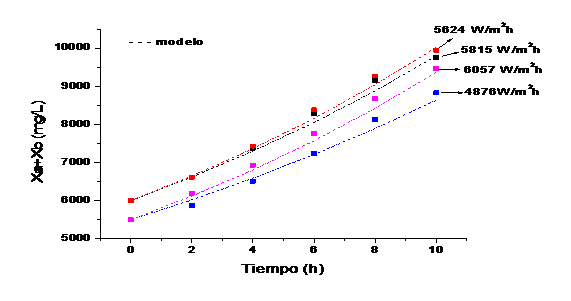
Fig. 5 Growth kinetics of microalgae and bacteria at different solar irradiation accumulated according to the model and the experimental data.
Conclusions
The obtained model allows to predict the maximum concentrations of the mixed biomass of chlorella vulgaris (11 gL-1) considering the effect of solar radiation accumulated in the day, which reaches its optimum value at 5209 W / m2h.
The validation of the model showed a high percentage of correlation (99,2) between the observations and the predictions, indicating a good performance in practice for variable solar irradiation conditions.