INTRODUCTION
Mayonnaise-type soy dressing is an oil-in-water emulsion found in Cuban markets which contains amounts of oil and therefore is highly sensitive to autoxidation and rancidity. It is a semi-solid emulsion, mostly of the O/W type, consisting of soybean oil, vinegar, seasonings, corn starch, soybean paste and potassium sorbate, which is obtained from the crushing the shelled soybean, which is hydrated, ground and pasteurized, until it reaches a homogeneous and pasty appearance. Its nutritional composition comprised 30% fat, 10.3% carbohydrates and 2.1% protein. Development and production of undesirable off-flavors (rancidity) and toxic compounds occur during oxidation of lipids has been recognized as the main cause of quality deterioration in food emulsions (Gorji et al., 2016).
The primary oxidation products of unsaturated fatty acids are the hydroperoxides, highly reactive compounds that decompose rapidly, yielding a complex mixture of non-volatile and volatile compounds such as hydrocarbons, alcohols, acids, aldehydes and ketones, able to affect the overall quality of the products (Gorji et al., 2016). Volatile lipid oxidation products, also referred to as secondary oxidation products, are responsible of flavor and taste deterioration in food.
Food industry has great interest on preventing of lipid oxidation, as well as on its early detection, in relation to the economical profits derived from the increase of consumers acceptance. Therefore, a marker that could assess the state of lipid oxidation, advising the products’ consumer, is of great interest (Frérot, 2017). Detection of lipid oxidation needs to be accomplished using a specific, sensitive and rapid method. A wide spectrum of lipid oxidation tests exists, ranging from simple organoleptic evaluation to more complex chemical methods, but most of them have lack of sensitivity and specificity (Laguerre et al., 2007).
Hexanal has become a well-known indicator, being a major product of fats oxidation, which rises during storage. This aldehyde has been determined in various food matrixes, using several sample preparation methods and detection techniques (Brunton et al., 2000; Goodridge et al., 2003; Sanches-Silva et al., 2004; Giuffrida et al., 2005; García-Llata et al., 2006; Kaykhaii & Rahmani, 2007; Panseri et al., 2011; Azarbad & Jeleń, 2015).
Solid-phase microextraction combined with static headspaces (HS-SPME) makes use of a fiber that adsorbs analytes from sample. It offers high throughput performance and does not require extended samples preparation, thus resulting in time saving. Moreover, it is reproducible, sencitive, solventless, simple and effective and eliminates interference compounds from the sample matrix with improvement in selectivity of the analysis (Xu et al., 2016).
The aim of this study was to develop and validate an HS-SPME-GC-MS method as useful procedure for quantifying hexanal in mayonnaise-type soy dressing.
MATERIALS AND METHODS
Sample and chemicals
The mayonnaise-type soy dressing with the commercial trademark Aurora was produced in the pilot plant of vegetables at the Food Industry Research Institute. Hexanal (99% purity), hexan-1-ol (99.9%) and a n-paraffin solution (C5-C24) were purchased from Sigma-Aldrich (Steinheim, Germany).
Headspace-solid phase microextraction
Three SPME fibers were tested: 75(m carboxen/polydimethylsiloxane coating (CAR/PDMS), 65(m polydimethylsiloxane/divinylbenzene coating (PDMS/DVB) and 50-30 (m divinylbenzene/carboxen/polydimethylsiloxane (DVB/CAR/PDMS) coating fibers. These fibers were obtained from Supelco (Bellefonte, PA, USA). HS-SPME was performed by incubating 0.3 g of soy dressing in an 8-mL Teflon-lined septum cap vial to 40 oC during 10 min, and then extracting for 40 min. Two samples with different contents of hexanal (25 and 50 ng/g) were analyzed.
A fresh soy dressing (10 ng/g hexanal content) was used for determining the main parameters affecting the HS-SPME efficiency: temperature and time of extraction. The lower and upper limits of temperature and extraction time were set at 30, 50 oC and 20, 40 min, respectively. The maximum temperature was 60 oC to avoid the oxidation of the product. Before extraction, 0.1 mL of an hexan-1-ol solution (1 (g/mL in methanol) was added to the vial as internal standard. Carry over and peaks originating from the fiber were regularly assessed by running blank samples and after each analysis fibers were maintained in the heated GC injector for additional 5 min to prevent contaminations.
Gas chromatography-mass spectrometry
GC-MS analysis was carried out using a Shimadzu QP-2010 Ultra (Kyoto, Japan), equipped with a 30 m x 0.25 mm i.d., 0.25 (m film thickness DB-5ms capillary column (J&W Scientific, Folsom, CA, USA). Carrier gas was helium at 1 mL/min. Oven temperature was held at 50 oC for 2 min, increased at 4 oC/min up to 70 oC and then at 20 oC/min until 250 oC. Thermal desorption was carried out at 300 oC for CAR/PDMS, 250 °C for PDMS/DVB and 270 °C for DVB/CAR/PDMS fiber. Injection was in splitless mode for 2 min, using and inlet liner of 0.75 mm i.d. The MS worked in electron impact mode at 70 eV ionization energy and in scanned mode from m/z 35 to 350, at 1.3 scan/s. Ion source and connecting parts temperature, 250 oC.
Identification of compounds was achieved by comparison of their linear retention index and mass spectra with those shown by reference standards. Linear retention indexes were calculated using a mixture of n-paraffins.
Two methods were used for quantification (Ouyang and Pawliszyn, 2008). The first, based on standard addition, was used to determine the initial amount in a fresh sample of soy dressing. Determinations were made on the selected sample and on two other samples to which 15 and 45 ng of hexanal were previously added. With the three points, the hexanal concentration of the fresh sample was calculated. Once the HS-SPME method was established, the hexanal concentration was determined in triplicate by the internal standard method using a relative area calibration curve (analyte area/internal standard area) vs. hexanal content. Hexanal standard solutions in methanol (0.3 mL), with increasing contents of the analyte (15 ng to 3000 ng/mL) and 0.1 mL of the internal standard solution were added to the fresh dressing to obtain the calibration curve. The amounts of hexanal added were 15, 40, 240, 990, 1990 and 2990 ng.
Validation of the method
The analytical parameters of the method (linearity, limit of detection (LOD), limit of quantification (LOQ), repeatability and accuracy) were determined to validate HS-SPME-GC-MS procedure for hexanal determination in mayonnaise-type soy dressing samples.
For linearity a calibration curve was obtained by subjecting same aliquots of a soy dressing sample added with different hexanal amounts and a constant quantity of the internal standard (100 ng) to the optimized procedure and by reporting hexanal area/internal standard area vs. hexanal content (ng/g). In the range 25-3000 ng. The detection and quantification limits of hexanal (LOD and LOQ) were estimated according to relationships LOD = 3⁄SDo/slope and LOQ = 10⁄ SDo/slope, where SDo: standard deviation of residues and b: equation intercept (FAO/WHO, 2011). Repeatability of the method was expressed as the relative standard deviation (RSD) obtained from the determination of eight different samples prepared by the addition of known amounts of hexanal at tree levels (25, 50 and 1000 ng/g). Accuracy was estimated by recovery assay. Eight soy dressing samples were spiked with three hexanal amounts (25, 50 and 1000 ng/g).
Statistical analysis
Optimization was made by response surface design (Montgomery, 2013). A three-level factorial design (32) was selected to assess the main effects and two factor interactions. Four center points per day (factorial design was realized in 4 days) were added to ensure enough degrees of freedom for block factor evaluation. Total number of experimental points needed were twelve. Numerical
optimization was used to find a point that maximizes the desirability function. Desirability is an objective function that ranges from zero outside of the limits to one at the goal. Once it hits one then, all goals have been completely satisfied. The Design-Expert 8.0.6 program (Stat-Ease Corp., Minneapolis, MN, USA) was used.
RESULTS AND DISCUSSION
Three types of SPME fibers (65 μm PDMS/DVB, 75 μm CAR/PDMS and 50/30 μm DVB/CAR/PDMS) were tested and compared in this study to determine which one is most efficient to extract hexanal. The comparison of the SPME fiber performance was made in terms of extraction efficiency and repeatability. Among the three SPME fibers tested (Fig. 1), CAR/PDMS fiber exhibited higher signal intensity in comparison to DVB/CAR/PDMS and PDMS/DVB fibers.
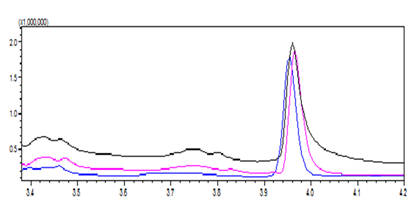
Fig. 1 Selected ion monitoring chromatogram showing hexanal isolated by the three fibers. CAR/PDMS (black), DVB/CAR/PDMS (violet), PDMS/DVB (blue).
The CAR/PDMS fiber extracted the highest amount of hexanal at the two concentrations of the aldehyde evaluated (Fig. 2), since the PDMS/DVB and DVB/CAR/PDMS fibers had approximately a 20% lower extraction efficiency. However, CAR/PDMS fiber was the one with the lowest repeatability (7.4 and 8.2% for 25 and 50 ng/g, respectively), while PDMS/DVB (2.6 and 3.3%) had higher repeatability. The relative standard deviation with PDMS/DVB fiber were less than 6.7% for hexanal in aqueous solutions (Song et al., 1998), but greater than 1.2% for hexanal in turkey muscle (Brunton et al., 2000).
Górecki et al. (1999), by modeling with the Langmuir isotherm, considered the surface of the porous polymer adsorbents as covered by a monomolecular layer that reaches equilibrium conditions quickly in the CAR/PDMS fiber. These authors also suggested that capillary condensation can occur in the small pores of the Carboxen, which would greatly inhibit reaching equilibrium under similar conditions with the consequent decrease in repeatability. This behavior with the CAR/PDMS fiber has also been reported by other authors (Kataoka et al., 2000; Brunton et al., 2000).
This fact, together with the fact that the longer useful life of the PDMS/DVB fiber is known with respect to CAR/PDMS, determined that the PDMS/DVB fiber was selected for the quantitative determination of hexanal in the mayonnaise-type soy dressing.
Once the fiber to be used has been defined, other important parameters that must be considered in the HS-SPME are stirring, temperature and extraction time (Balasubramanian & Panigrahi, 2011). Stirring was not considered to simplify the procedure, since Iglesias et al. (2007) showed that with small amounts of oil-water emulsions (up to 1 g) it was not an important parameter.
Therefore, a factorial design was made to optimize the extraction temperature and time. A quadratic polynomial model was fitted on generated data of microextraction process (Fig. 3); this model can predict response at every point, even those that have not been placed in the design. The ANOVA confirmed acceptability and qualification of the regression model which had excellent ability in predicting responses by considering very low probability (p value <0.001) of the F value 28.31. The “lack of fit (LOF) p value” of 0.357 indicates that the LOF is not significant relative to the pure error. Also, the coefficient of determination (R2 = 0.959) was very high.
Based on results of the optimization experiments, the optimum conditions for extraction of hexanal from mayonnaise-type soy dressing samples were selected as follows: extraction temperature of 40 °C and extraction time 35 min. At this point, the desirability function hits 0.978 from a maximum value of one.
Considering that in the analysis by SPME interferences in the determination of the analyte can occur because of the composition of the matrix, which is known as the matrix effect (Souza-Silva et al., 2015), it was necessary to apply some method as the internal standard that eliminate this interference. Therefore, it was essential to know the concentration of the analyte in the fresh soy dressing. For this purpose, the standard additions method was used. The coefficient of determination was high (R2 = 0.995) and interpolation to zero addition of hexanal resulted in a fresh dressing content of 10 ng/g. This value was considered to continue the validation of the analytical method.
The analytical parameters of the method (linearity, LOD, LOQ, repeatability and accuracy) were determined to validate HS-SPME-GC-MS procedure. The calibration curve obtained by subjecting same aliquots of a soy dressing sample added with different hexanal amounts (Fig. 4) had the equation:
Hexanal area/Internal standard area = 0.015 Hexanal content + 0.031
The residual standard deviation (SDo) of 0.005 and a correlation coefficient of 0.9998, confirming the suitability of PDMS/DVB fiber to monitor hexanal content both in fresh and oxidized mayonnaise-type soy dressing samples with excellent linearity.
The LOD and LOQ of hexanal resulted 1.06 ng/g and 3.53 ng/g, respectively. The LOD was lower than the values reported by other authors using HS-SPME-CG-MS method (Garcia-Llatas et al., 2006; Mallia et al., 2008) and similar to those found by Sanches-Silva et al. (2004) and Panseri et al. (2011) for other food products. The LOQ was higher than 1,6 ng/g of hexanal in beef bouillons determined with DVB/CAR/PDMS (Giuffrida et al., 2005), but similar to 3,40 ng/g in butter determined with CAR/PDMS (Panseri y col., 2011) y less than 270 ng/g of hexanal using static headspace analysis (Azarbad & Jeleń, 2015).
The repeatability was determined by the analysis under similar conditions (same analyst, equipment and short time interval) of eight samples with three levels of hexanal (Table 1). The mean repeatability was 3.3%, much lower than the 10% reported by Iglesias et al. (2007) for hexanal in mayonnaise using CAR/PDMS; 4.9% in butter with CAR/PDMS (Panseri et al., 2011); 18.7% reported in beef broth with DVB/CAR/PDMS (Giuffrida et al., 2005); 5.3% in walnuts using CAR/PDMS (Pastorelli et al., 2007) and 5.4% in mayonnaise with the use of static headspace analysis (Azarbad & Jeleń, 2015). Besides this, Goodridge et al. (2003) reported repeatabilities between 1 and 13% with the 100 µm PDMS fiber in chicken myofibrils.
Recovery was determined by observing several measurements made at three levels of hexanal, considering the concentration range of the calibration curve (Table 1) presents the recovery percentages that were between 97.3 and 98.0%, which can be considered as good. The mean recovery was 97.7%, which is within the established interval of 95 to 105% for a level of 0.1% and from 97 to 103% for a level of 1%, according to the (AOAC, 2005) and (Codex Alimentarius, 2010), for which the value obtained is considered acceptable for the level measured in the samples. In agreement with other similar studies, Giuffrida et al. (2005) reported between 85 and 88% for hexanal in beef broth with DVB/CAR/PDMS, Iglesias et al. (2007) reported recoveries between 80 and 120% in mayonnaise with the CAR/PDMS fiber and Panseri et al. (2011) obtained 97.37% in butter with the CAR/PDMS fiber.
RSD: relative standard deviation. n = 8
The optimized method was used to evaluate the suitability of hexanal as indicator of lipid oxidation process during the storage in term of oxidation stability (Fig. 5). Results showed a linear constant increasing of hexanal reaching the maximum level of 32.9 ng/g at 60 days of storage at 15 oC.
CONCLUSIONS
A method was established by headspace-solid phase microextraction (HS-SPME) procedure combined with gas chromatography for the determination of hexanal as an indicator of the oxidative changes in mayonnaise-type soy dressing with the polydimethylsiloxane-divinylbenzene fiber (PDMS/DVB), 0.3 g of sample, 10 min of pre-extraction, 35 min of extraction at 40 oC and 2 min of desorption at 250 oC. Validation for the hexanal content technique showed that the method has a linear response in the range of 1 to 3000 ng/g of hexanal, has a detection limit of 1.06 ng/g and a limit of quantification of 3.53 ng/g, has an average repeatability of 3.3% and has 97.7% recovery.