Meu SciELO
Serviços Personalizados
Artigo
Indicadores
-
Citado por SciELO
Links relacionados
-
Similares em SciELO
Compartilhar
Cultivos Tropicales
versão On-line ISSN 1819-4087
cultrop vol.40 no.1 La Habana ene.-mar. 2019
Original Article
Response of soybean (Glycine max (L) Merr) to inoculation with Azospirillum and Bradyrhizobium
1Instituto de Investigaciones de la Caña de Azúcar (INICA), carretera CUJAE Km 1½, Rancho Boyeros, CP 19390, La Habana, Cuba
2Instituto de Ciencias Agrarias (ICA-CSIC), Consejo Superior de Investigaciones Científicas (CSIC), Serrano 115, dpdo., CP 28006 Madrid, España
Azospirillum brasilense is a nitrogen-fixing plant growth promoting rhizobacterium with potential as biofertiliser for leguminous and non-leguminous plants. Agriculturally important crops, especially sugar cane, have been often inoculated with A. brasilense producing increases in growth parameters and yield in different soils and climatic regions. Sugar cane inoculated with A. brasilense has been grown in intercropping with legumes like beans and soybean. In field experiments of intercropping of sugarcane inoculated with A. brasilense and soybean carried out in Cuban soils, an increase in most of the growth parameters of sugarcane have been observed, but it is not known the effects produced on the other intercropped plant. This work analyzes the alterations in plant and nodule development, nitrogenase activity and colonization of roots by Azospirillum, in soybean inoculated with A. brasilense 8-INICA and Bradyrhizobium japonicum USDA-110. A. brasilense inoculation did not produced changes in the height and biomass of aerial parts of the plant or in nitrogenase activity in nodules. However increases in root and nodule biomass were detected with its inoculation. A. brasilense colonized root surface by adhesion to mucilaginous material where bacteria proliferate and are able to form microcolonies inside. The break caused by the emergence of a secondary root allowed the bacterium to enter in the intercellular spaces of plant root parenchyma and between epidermal root cells, allowing soybean root colonization. These results suggested that A. brasilense 8-INICA can live as an endophyte in soybean roots and could be a suitable candidate like inoculant of leguminous with agricultural purposes.
Key words: leguminous; PGPRs; bacterial inoculants; endophytes
INTRODUCTION
The importance of increasing crop yields in subsistence farming systems characterized by a minimal use of mineral fertilizers goes through improve beneficial microorganisms-root-soil interactions leading to higher crop yields 1.The effect on legumes of co-inoculation with nitrogen-fixing symbiotic bacteria and non-symbiotic plant growth promoting genera (PGPRs) is gaining increasing interest within the current biological nitrogen fixation and has been proposed like a new biotechnological tool to improve yield and sustainability 2.
A. brasilense is a nitrogen-fixing plant growth promoting rhizobacterium (PGPR) with a substantial potential as biofertilizer for leguminous and non-leguminous plants. Agriculturally important crops, especially sugar cane, have been often inoculated with A. brasilense producing increase in growth parameters and yield in different soils and climatic regions 3. Different mechanisms of action have been proposed to explain the enhancement of plant growth, like proliferation of the root system 4.The stimulation of growth is usually due to the production of phytohormones such as auxins, cytokinins, gibberellins 5,6. Furthermore induction of plant-stress tolerance and defense genes has been described 7.
The combined application of bacteria of the genus Rhizobium or Bradyrhizobium with Azospirillum, produced a positive effect on legumes 8. The positive effect results in early nodulation, increase in nodule numbers, nitrogenase activity and root respiration, as well as an overall improvement in the uptake of water and nutrients by the roots. Azospirillum PGPR characteristics may be the reason for its effect on legume co-inoculations, which would be related to the induction of a greater number of infective root hairs, differentiated from epidermal cells and an increase in the secretion of flavonoids inducing nod genes 9.
Intercropping is a sustainable method for crop production used to maximize utilization of available resources and could improve biodiversity of soils. In tropical soils it has been proven that the intercropping of grasses with legumes, represents a group of advantages among which we can point out the contribution of nitrogen to the soil and the obtaining of additional productions of fiber, fat and food 10. Furthermore, intercropping improves resilience, food security 11 and nutrition.
In field experiments of intercropping of sugarcane inoculated with A. brasilense and not-inoculated soybean, carried out in Cuban soils 10, increases in most of the growth parameters of sugarcane have been observed, but it is not known the effects produced by Azospirillum inoculation on the other intercropped plant. The aim of this work was to evaluate, under controlled conditions, the effect of A. brasilense 8-INICA and B. japonicum USDA-110 by separated and co-inoculation of the two microorganisms in plant height, shoot and root fresh weight, nodule dry weight and nitrogenase activity in nodules of soybean roots (Glycine max (L) Merr), emphasizing in soybean root colonization by Azospirillum.
MATERIALS AND METHODS
Strains
A. brasilense 8-INICA strain isolated from sugarcane cv. Ja 60-5 rhizosphere in Ciego de Ávila province, Cuba 12 cultured in NFb medium at 28 ºC, 100 rpm and maintained in BMS broth 13 containing 20 % (v/v) glycerol at -80 ºC. B. japonicum USDA-110 14 donned by Centro de Investigación y Formación Agraria Las Torres-Tomejil (Sevilla, Spain), grown and maintained in Vincent medium 15.
Plant growth and nitrogenase activity
Soybean (Glycine max cv. Williams) seeds were surface sterilized in 0.1 % HgCl2 for 5 min and then washed several times with sterile distilled water. Seeds were sown in 1 L autoclaved Leonard jars filled with sterile vermiculite and inoculated with 1 mL of A. brasilense 8-INICA or B. japonicum USDA-110 suspension containing 108 colony forming units (CFU mL-1). Treatments were: Nitrogen (control treatment) non-inoculated plants and watered with a solution containing 35 ppm of nitrogen in form of potassium nitrate, plants inoculated only with A. brasilense 8-INICA, plants inoculated only with B. japonicum USDA-110 and plants inoculated with the two microorganisms. The inoculations treatments were watered with a free nitrogen nutritive solution. Nutrive solutions were replaced three times per week. The conditions of the growth chamber were: a 16-h-light/8-h-dark photoperiod, 25 ºC day/15 ºC night temperature, 58 % relative humidity and an irradiance level of 200 μm m-2 s-1. Three replicates were used for inoculated and non-inoculated plants. After five weeks plants were harvested for plant growth parameter determinations and microscopy purposes. It was determined plant height, shoot and root fresh weight, nodule dry weight and nitrogenase activity in nodules. Nitrogenase activity was measured by the acetylene reduction assay (ARA) on nodulated roots from plants inoculated with Bradyrhizobium and co-inoculated with Bradyrhizobium + Azospirillum, following the method described by other authors 16.
Processing of sampling for microscopy
Antibodies against Azospirillum were obtained in order to confirm that the strain of Azospirillum inoculated was the same that those that was found in soy roots. A. brasilense 8-INICA strain was cultivated as indicated above for 24 h, in a known volume of BMS medium. Cells were harvested and sterile formaldehyde was added to the pellet to achieve a final concentration of 5 per 1000 and left overnight at 27 ºC. Cells were centrifuged at 5000 rpm for 5 min. The pellet was washed with sterile phosphate buffer saline pH 7.2 (PBS) and suspended in sterile PBS pH 7 until reaching109 cell mL-1, measured by optical density at 680 nm. The final pellets were suspended in equal quantities of Freund's complete adjuvant. Antiserum was obtained in white rabbits by intravenous immunization route, one injection every eight days for five weeks. Blood was collected eight days after the last injection. The final bleed-out serum from each rabbit was collected by centrifuging clotted blood at 2.000 g and stored at -20 ºC. Preimmunization sera were obtained from each rabbit.
Processing of sampling for microscopy
Only plants inoculated with Azospirillum were processed for microscopy. In order to prove the specificity of the antiserum, Azospirillumin in vitro cultures were embedded first in agar 17.The agar pieces were processed as root samples to be embedded in LR-White resin 18. Small pieces of fresh roots were selected for a comparable stage of development. Samples were taken at harvest time, cut with a razor blade, fixed in 2.5 % (v/v) glutaraldehyde in 50 mM Na-cacodylate, pH 7.4 and vacuum-infiltrated to enhance penetration of the fixative. Dehydration was performed using an ethanol series and nodules pieces were infiltrated and finally embedded in LR-White Resin (London Resin Corporation) by heat polymerization at 60 °C for 24 h in gelatin capsules 19. Semi-thick (1 μm) and ultrathin (70 nm-thick) sections were cut in a Reichert Ultracut S ultramicrotome for light microscopy, and electron microscopy, respectively. The semi-thick sections were stained with 1 % (w/v) toluidine blue in aqueous sodium borate (1 %) for direct examination using a Zeiss Axiophot photomicroscope.
Light and electron microscopy: immunocytochemistry and silver enhancement
For immunocytochemistry and silver enhancement, glass slides with 1 µm thick sections were placed at 37 ºC, overnight to ensure adhesion of sections. Sections were thoroughly washed in PBS, pH 7.2, containing 0.01 % pure gelatin, 0.1 % BSA, and 0.05 % sodium azide. Blocking of unspecific binding sites was achieved by incubation of sections in the above solution containing 20 mg of Bovine Serum Albumine (BSA) per mL, for 20 min at room temperature. Incubation in primary antibodies (anti-A. brasilense 8-INICA) diluted 1:500 in the same buffer, was carried out for 1 h in oven at 37 ºC. Sections were then rinsed in PBS and the excess of buffer gently removed. Incubation with goat anti-rabbit gold conjugate (GAR 15 nm; BioCell, Cardiff, U.K.) diluted 1:40 in PBS was performed for 1 h at room temperature. Sections were then rinsed in PBS and distilled water. Silver enhancement was carried out with IntenSE M Silver Enhancement Kit (Amersham, Buckinghamshire, U.K.) and allowed to progress while monitored under the microscope 19. Sections were then energetically rinsed in tap and distilled water, counterstained with 0.05 % (w/v) basic fuchsine (Carlo Erba, Milano, Italy) in 5 % (v/v) aqueous ethanol, rinsed and let to dry. Sections were then mounted and photographed under a Zeiss Axiophot photomicroscope. For electron microscopy, sections (70 nm thick) were collected on nickel grids and immunolabelled 18. Counterstaining of sections was obtained with 2 % aqueous uranyl acetate (5 min) and lead citrate for 2 min. After rinsing and air-drying, sections were examined using a STEM LEO 910 electron microscope at an accelerating voltage of 80 kV.
RESULTS AND DISCUSSION
The ability of A. brasilense to stimulate plant growth has been extensively demonstrated 5,7. Previous reports have shown that co-inoculation of Rhizobium and Azospirillum enhanced the nodulation and yield of leguminous plants 2. In our results, A. brasilense 8-INICA inoculation did not produced changes in the height and biomass of aerial parts of the plant nor in nitrogenase activity (Table 1). However an increase of 61.8 % in root weight with respect to control was observed in plants inoculated only with Azospirillum, while an increase of only 6 % was observed in plants inoculated only with Bradyrhizobium. Nevertheless co-inoculation produced an increase of 11 % in this parameter. An increase in nodule weight of 78.9 % was observed in co-inoculated plants with respect to plants inoculated only with Bradyrhizobium. Since the increase in the weight of the nodules did not translate into an increase in nitrogenase activity, this increase cannot be understood as an increase in the number of bacteroids, but rather in the vegetable tissue of the nodule. Plants treated only with nitrogen or A. brasilense 8-INICA respectively did not produce nodules. The increase in root and nodule weight with Azospirillum inoculation was consistent with others results in Phaseolus vulgaris9 inoculated with Rhizobium tropici and R. etli and the same species of Azospirillum. Improvement of root growth can be produced by enhanced uptake of minerals and water 20, thus, Azospirillum inoculations of soybean could represent an advantage in crop yield.
Table 1 Effects of A. brasilense 8-INICA and B. japonicum USDA-110 and co-inoculation of the two microorganisms on soybean growth parameters and nitrogenase activity
Treatments | Height (cm) | Shoot FW (g) | Root FW (g) | Nodules DW (g) | Nitrogenase activity (µmol ethylene g nodule-1 h-1) |
---|---|---|---|---|---|
Nitrogen | 38.55±0.96a | 1.48±0.13a | 3.82±0.54b | nn | - |
|
38.94±3.03a | 1.40±0.16a | 6.18±0.32a | nn | - |
|
41.29±2.59a | 1.45±0.15a | 4.05±0.58b | 0.019±0.003b | 8.81±1.053a |
|
42.40±1.73a | 1.13±0.19a | 4.26±0.54b | 0.034±0.006a | 7.96±1.19a |
Legend: FW (Fresh Weight), DW (Dry Weight) and nn (no nodules)
A.b+B.j: co-inoculation of Azospirillum and Bradyrhizobium
Azospirillum and Bradyrhizobium treatments were watered with a nitrogen free nutritive solution and with a solution containing 35 ppm in the nitrogen treatment. Data are means followed by standard error of three repetitions. Means followed by the same letter within a column are not significantly different. Statistical data were obtained by SPSS.
Soybean roots colonization by Azospirillum
The establishment of efficient bacterial populations in the rhizosphere is essential to improve root colonization and plant productivity 21. Soybean root colonization was studied at the end of plant culture in plants inoculated only with Azospirillum, using light and electron microscopy and immunocytochemistry. A. brasilense8-INICA colonized root surface by adhesion to mucilaginous material produced by the plant where bacteria proliferate (Figure 1 and Figure 2A and B).
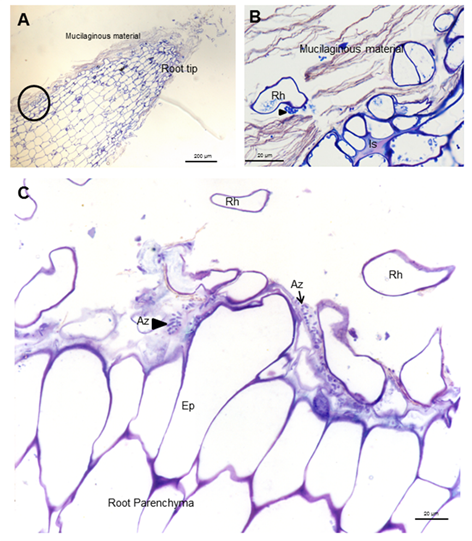
Figure 1 Light micrographs of longitudinal and transverse sections showing A. Brasilense 8-INICA, surface colonization five weeks after inoculation, stained with toluidine blue
Furthermore, carbohydrates produced by the bacteria can be observed. Carbohydrates were detected by fuchsine staining 19. (Figure 1C and Figure 2B and C).
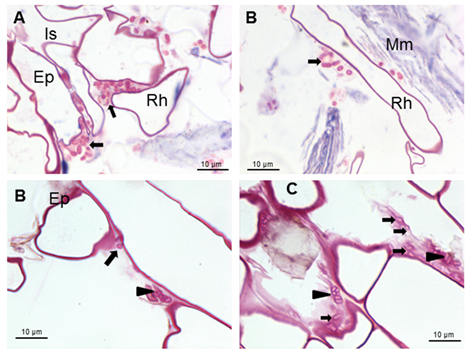
Figure 2 Light micrographs of longitudinal sections showing Azospirillum brasilense 8-INICA surface colonization five weeks after inoculation, stained with fuchsine
These carbohydrates could be able to confer protection to the root against external conditions and may favor colonization of the bacterium in its natural habitat 22. Results show bacteria very close to root hairs near the radicular tip (Figure 1A and B). Microcolonies are observed on the root surface embedded in a self-produced carbohydrate polymer matrix which was consistent 23, as shown in figures 2B and C (arrowheads) and under protection of the root hair (Figures 2A and C). This gives rise to biofilm, which is a mechanism that allows bacteria to live in extreme or changing conditions and one of the most widespread used in competitive root colonization of efficient bacteria. After surface root colonization, the intercellular parenchymatic colonization is produced. Azospirillum was able to take the break caused by the emergence of asecondary root (Figures 3A and B) and enter in the intercellular spaces of plant root parenchyma (Figures 3A and C) and between epidermal root cells allowing the colonization. Root morphology changed as a consequence of this colonization, producing a matrix between two layers of parenchyma root cells (Figures 3B and D).
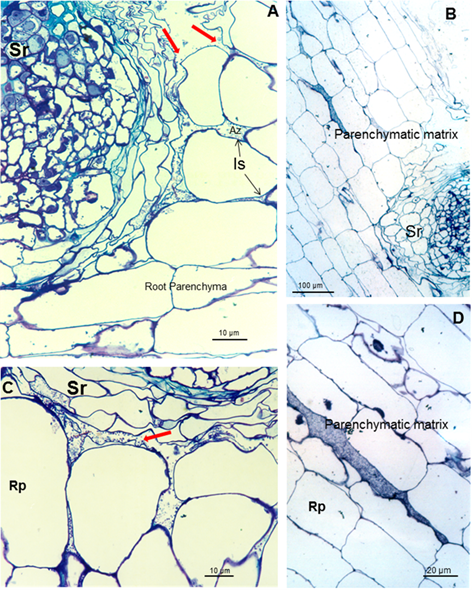
Figure 3 Light micrographs of longitudinal and transverse sections of soybean roots inoculated with A. brasilense 8-INICA showing root colonization after five weeks of inoculation (A-C); colonization of soybean roots through the gaps produced by the emergence of a secondary root and D the intercellular spaces of inner layers of cortical cells
This parenchymatic matrix facilitates bacterial growth and the low oxygen partial pressures for the expression of nitrogenase and can directly contribute to nitrogen to the plant and the exchange of nutrients between the bacteria and the plant is assured. Soil and rhizosphere bacteria can affect the mineral nutrition of plants by changing root-uptake characteristics, due to a modification of root morphology or alteration of uptake mechanisms, relative growth rate or internal composition of plants 24). The fact to survive inside the root and not producing any injuries confirm this microorganism as an endophytic bacterium 25. Endophytes can be helpful in protecting the plant from environmental stress as well as in supplying the plant with nitrogen and other growth promoting substances 26.
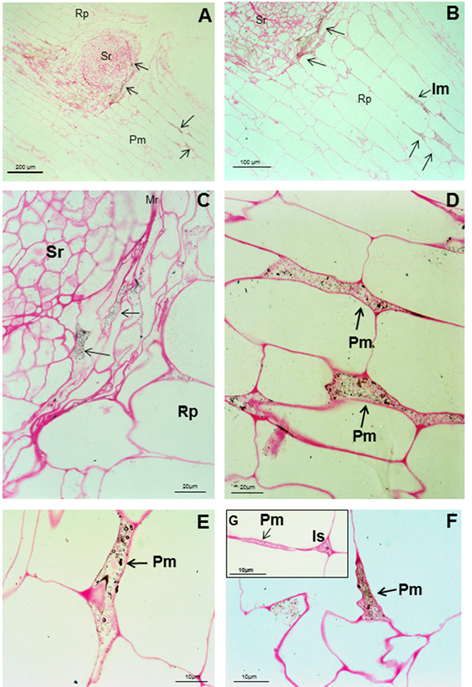
Figure 4 Light micrographs of longitudinal and transverse sections through soybean root five weeks after seed inoculation with A. brasilense 8-INICA
Anti-A. brasilense 8-INICA reactions with the microorganism, showed, at light microscopy, the bacteria surrounded by gold particles (Figure 4). Immunolocalization and silver enhancement demonstrates that colonization by A. brasilense 8-INICA is achieved, knowing that the bacteria are labelled in the break caused by the emergence of a secondary root (Figure 4A-C), in the intercellular spaces (Figure 4D) and in the parenchyma matrix produced by Azospirillum colonization of plant root parenchyma (Figure 4D-F). With the high magnification at light microscopy (Figure 4G) it can be observed that the intercellular spaces and the parenchymatic matrix are connected, and Azospirillum identified by the antibody can be seen as bodies surrounded by a black circle. These results in inoculation experiments in soybean firmly show colonization by Azospirillum which is consistent with similar reports on rice 27, tobacco 28, tomato 29 and barley 30. In the case of sugarcane, the controversy of whether it is only a rhizospheric or a root associated bacterium or an endophyte requires further studies leading to clarify this aspect which could be influenced by quorum sensing3 and other factors. Immunolocalization in ultrathin slides of Azospirillum cells embedded in agar with the antibody anti-A. brasilense 8-INICA shows an enormous affinity of the antibody to the antigen (Figure 5A). There is intense labeling of the bacteria with 15 nm gold particles. The number of gold particles is elevated and marked the different layers of the cellular envelopes (Figure 5A, arrows).
The number of gold particles decreases considerably when the marking is done in the intercellular spaces, as if the bacteria would lose some of the layers with the colonization of the root parenchyma (Figure 5B), although still labeling in different layers can also be observed (arrows). Inside the parenchymatic matrix only one layer is labeled (Figure 5C and D) and no gold particles are detected inside the bacteria. Unspecific labeling can be observed in vesicles of the parenchymatic matrix, probably formed by bacterium membranes degradation (Figure 5C).
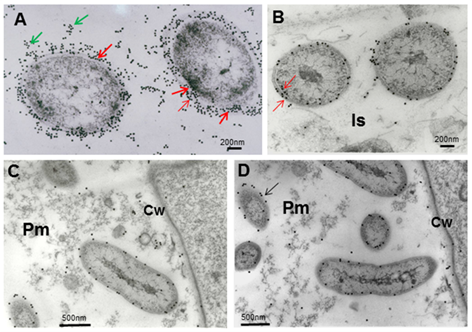
Figure 5 Electron micrographs showing immunolocalization of A. brasilense 8-INICA epitopes growing in culture medium (A) and colonizing the intercellular spaces and the parenchyma of soybean roots (B-D)
When the bacterium ages, the cytoplasm is retracted and the gold particle remains on the outermost layer of the bacterium envelopes (Figure 6A and B). Although not normal, in some areas appear gold particles on the cell wall (Figure 6C). Very few gold particles appear in the cytoplasm of adjacent cells to the matrix. There is no localization of gold particles in aged organelles (Figure 6D).
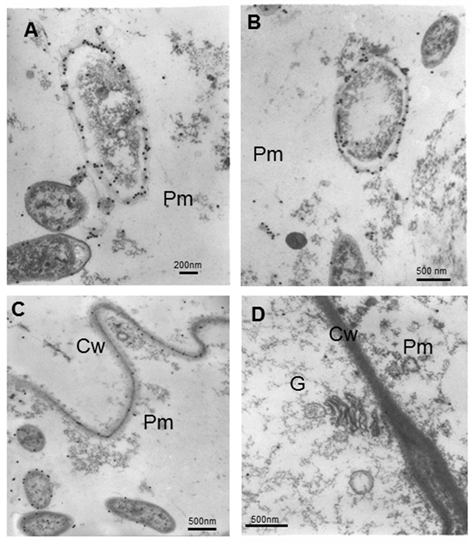
Figure 6 Electron micrographs showing immunolocalization of A. brasilense 8-INICA, colonizing the intercellular spaces and the parenchyma of soybean roots embedded in LR-White resin
Azospirillum is usually associated with roots of grasses such as sugar cane, rice and maize 4, translating benefits to the plants for its contribution to nutrition. Microscopy techniques and immunolocalization allowed to confirm in this work that A. brasilense 8-INICA can act as an endophyte in soybean roots. Endophytes can be helpful in protecting the plant from environmental stress as well as in supplying the plant with nitrogen and other growth promoting substances 26. This work emphasizes that Azospirillum is not only interesting for sugarcane inoculation, but also for soybean and therefore would work very well in intercropping of soybean and sugarcane, with the advantage of not having to introduce foreign strains in Cuban soils.
CONCLUSIONS
A. brasilense 8-INICA is able to colonize soybean roots, not only superficially but also intercellularly forming a parenchymatic matrix which favors the exchange between the bacterium and the plant, making A. brasilense 8-INICA an endophyte of soybean roots. Besides its enhanced effects on root and nodule weight in comparison with nodulating species like B. japonicum USDA-110, which confirm reports of other researchers, this result of being an endophyte in soybean roots is important in order to accomplish sustainability in agriculture, considering that field inoculations are environmental friendly and an economical feasible way to obtain good productions by means of organic agricultural practices.
Further field studies under non-controlled conditions must be carried out with this strain in order to explore its potential as a biofertilizer in intercropping practices with this leguminous and sugarcane. Is important to emphasize that A. brasilense 8-INICA is fit to Cuban soils conditions which avoids foreign strains to be introduced in Cuban soil environments.
ACKNOWLEDGEMENTS
This work was done thanks to the AECID projects, A / 8500/07 and A / 019119/08, and Ministerio de Economía y Competitividad AGL2013-40758-R, developed between ICA-CSIC (Madrid, Spain) and INICA (Havana, Cuba).
REFERENCES
1. Souza R de, Ambrosini A, Passaglia LMP. Plant growth-promoting bacteria as inoculants in agricultural soils. Genetics and Molecular Biology. 2015;38(4):401-19. doi:10.1590/S1415-475738420150053 [ Links ]
2. Marks BB, Megías M, Nogueira MA, Hungria M. Biotechnological potential of rhizobial metabolites to enhance the performance of Bradyrhizobium spp. and Azospirillum brasilense inoculants with soybean and maize. AMB Express. 2013;3(1):21. doi:10.1186/2191-0855-3-21 [ Links ]
3. Fukami J, Abrantes JLF, del Cerro P, Nogueira MA, Ollero FJ, Megías M, et al. Revealing strategies of quorum sensing in Azospirillum brasilense strains Ab-V5 and Ab-V6. Archives of Microbiology. 2018;200(1):47-56. doi:10.1007/s00203-017-1422-x [ Links ]
4. Vital L, Mendoza HA. Azospirillum: Habitante de las Gramíneas. Revista: La ciencia y el hombre. 2014;XXVII(2):24. [ Links ]
5. Cassán F, Vanderleyden J, Spaepen S. Physiological and agronomical aspects of phytohormone production by model plant-growth-promoting rhizobacteria (PGPR) belonging to the genus Azospirillum. Journal of Plant Growth Regulation. 2014;33(2):440-59. doi:10.1007/s00344-013-9362-4 [ Links ]
6. Spaepen S, Bossuyt S, Engelen K, Marchal K, Vanderleyden J. Phenotypical and molecular responses of Arabidopsis thaliana roots as a result of inoculation with the auxin-producing bacterium Azospirillum brasilense. New Phytologist. 2014;201(3):850-61. doi:10.1111/nph.12590 [ Links ]
7. Fukami J, Ollero FJ, Megías M, Hungria M. Phytohormones and induction of plant-stress tolerance and defense genes by seed and foliar inoculation with Azospirillum brasilense cells and metabolites promote maize growth. AMB Express. 2017;7(1):153. doi:10.1186/s13568-017-0453-7 [ Links ]
8. Hungria M, Nogueira MA, Araujo RS. Soybean seed co-inoculation with Bradyrhizobium spp. and Azospirillum brasilense: a new biotechnological tool to improve yield and sustainability. American Journal of Plant Sciences. 2015;06(06):811-7. doi:10.4236/ajps.2015.66087 [ Links ]
9. Burdman S, Kigel J, Okon Y. Effects of Azospirillum brasilense on nodulation and growth of common bean (Phaseolus vulgaris L.). Soil Biology and Biochemistry. 1997;29(5-6):923-9. doi:10.1016/S0038-0717(96)00222-2 [ Links ]
10. Casas M, de Felipe M, Roldós J, Fernández-Pascual M, Guasch L. Co-inoculaciones de plantas de soja con bacterias de los géneros Rhizobium, Bradyrhizobium, Sinorhizobium, Azospirillum y Bacillus. In: Arrese-Igor C, Hernando MR, Tejo PMA, editors. Perspectivas de la fijación biológica de nitrógeno en el umbral del siglo XXI. España: Universidad Pública de Navarra; 1998. p. 193-4. [ Links ]
11. Chimonyo VGP, Modi AT, Mabhaudhi T. Perspective on crop modelling in the management of intercropping systems. Archives of Agronomy and Soil Science. 2015;61(11):1511-29. doi:10.1080/03650340.2015.1017816 [ Links ]
12. Pérez J, Casas M. Estudio de la interacción planta-Azospirillum en el cultivo caña de azúcar (Saccharum sp.). Cultivos Tropicales. 2005;26(4):13-9. [ Links ]
13. Baldani JI, Reis VM, Videira SS, Boddey LH, Baldani VLD. The art of isolating nitrogen-fixing bacteria from non-leguminous plants using N-free semi-solid media: a practical guide for microbiologists. Plant and Soil. 2014;384(1-2):413-31. doi:10.1007/s11104-014-2186-6 [ Links ]
14. Chibeba AM, Kyei-Boahen S, Guimarães M de F, Nogueira MA, Hungria M. Feasibility of transference of inoculation-related technologies: A case study of evaluation of soybean rhizobial strains under the agro-climatic conditions of Brazil and Mozambique. Agriculture, Ecosystems & Environment. 2018;261:230-40. doi:10.1016/j.agee.2017.06.037 [ Links ]
15. Vincent JM. A manual for the practical study of root-nodule bacteria [Internet]. Edimburgo, Escocia: Oxford?: [Published for the] International Biological Programme [by] Blackwell Scientific; 1970 [cited 2019 Jan 30]. 164 p. Available from: https://trove.nla.gov.au/version/4524921615. [ Links ]
16. Fernández-Pascual M, Pozuelo JM, Serra MT, De Felipe MR. Effects of cyanazine and linuron on chloroplast development, nodule activity and protein metabolism in Lupinus albus L. Journal of plant physiology. 1988;133(3):288-94. [ Links ]
17. Lucas MM, Vivo A, Pozuelo JM. Application of immunolabelling techniques to Bradyrhizobium dual occupation in Lupinus nodules. Journal of Plant Physiology. 1992;140(1):84-91. doi:10.1016/S0176-1617(11)81062-X [ Links ]
18. de María N, de Felipe MR, Fernández-Pascual M. Alterations induced by glyphosate on lupin photosynthetic apparatus and nodule ultrastructure and some oxygen diffusion related proteins. Plant Physiology and Biochemistry. 2005;43(10-11):985-96. doi:10.1016/j.plaphy.2005.09.001 [ Links ]
19. de Lorenzo CA, Fernández-Pascual MM, de Felipe MR. Subcellular localization of glycoprotein epitopes during the development of lupin root nodules. Protoplasma. 1998;201(1-2):71-84. doi:10.1007/BF01280713 [ Links ]
20. Bashan Y, de-Bashan LE. How the plant growth-promoting bacterium Azospirillum promotes plant growth-a critical assessment. Advances in Agronomy. 2010;108:77-136. doi:10.1016/S0065-2113(10)08002-8 [ Links ]
21. Jha PN, Gupta G, Jha P, Mehrotra R. Association of rhizospheric/endophytic bacteria with plants: a potential gateway to sustainable agriculture. Greener Journal of Agricultural Sciences. 2013;3(2):73-84. doi:10.15580/GJAS.2013.2.010313354 [ Links ]
22. Zivkovic M, Miljkovic M, Ruas-Madiedo P, Strahinic I, Tolinacki M, Golic N, et al. Exopolysaccharide production and ropy phenotype are determined by two gene clusters in putative probiotic strain Lactobacillus paraplantarum BGCG11. Applied and Environmental Microbiology. 2015;81(4):1387-96. doi:10.1128/AEM.03028-14 [ Links ]
23. Flemming H-C, Wingender J, Szewzyk U, Steinberg P, Rice SA, Kjelleberg S. Biofilms: an emergent form of bacterial life. Nature Reviews Microbiology. 2016;14(9):563-75. doi:10.1038/nrmicro.2016.94 [ Links ]
24. Xiaohui FAN, Zhang S, Xiaodan MO, Yuncong LI, Yuqing FU, Zhiguang LIU. Effects of plant growth-promoting rhizobacteria and N source on plant growth and N and P uptake by tomato grown on calcareous soils. Pedosphere. 2017;27(6):1027-36. doi:10.1016/s1002-0160(17)60379-5 [ Links ]
25. Reinhold-Hurek B, Hurek T. Life in grasses: diazotrophic endophytes. Trends in Microbiology. 1998;6(4):139-44. doi:10.1016/S0966-842X(98)01229-3 [ Links ]
26. Waqas M, Kim Y-H, Khan AL, Shahzad R, Asaf S, Hamayun M, et al. Additive effects due to biochar and endophyte application enable soybean to enhance nutrient uptake and modulate nutritional parameters. Journal of Zhejiang University-SCIENCE B. 2017;18(2):109-24. doi:10.1631/jzus.B1500262 [ Links ]
27. Rariz Mollo G, Ferrando L, Fernandez Scavino A. Aislamiento de bacterias endófitas fijadoras de nitrógeno en plantas de arroz cultivadas en diferentes suelos. In: VII Congreso de Medio Ambiente. La Plata, Argentina: Asociación de Universidades Grupo Montevideo; 2012. p. 20. Available from: http://hdl.handle.net/10915/2638727. [ Links ]
28. Chi F, Shen S-H, Chen S-F, Jing Y-X. Migration of Azospirillum brasilense Yu62 from root to stem and leaves inside rice and tobacco plants. ACTA BOTANICA SINICA. 2004;46(9):1065-70. [ Links ]
29. Fujita M, Kusajima M, Okumura Y, Nakajima M, Minamisawa K, Nakashita H. Effects of colonization of a bacterial endophyte, Azospirillum sp. B510, on disease resistance in tomato. Bioscience, Biotechnology, and Biochemistry. 2017;81(8):1657-62. doi:10.1080/09168451.2017.1329621 [ Links ]
30. Zawoznik M, Vázquez S, Díaz Herrera SM, Groppa MD. Search for endophytic diazotrophs in barley seeds. Brazilian Journal of Microbiology. 2014;45(2):621-5. doi:10.1590/S1517-83822014000200033 [/refs] [ Links ]
Received: May 08, 2018; Accepted: January 16, 2019