Meu SciELO
Serviços Personalizados
Artigo
Indicadores
-
Citado por SciELO
Links relacionados
-
Similares em SciELO
Compartilhar
Cultivos Tropicales
versão On-line ISSN 1819-4087
cultrop vol.42 no.3 La Habana jul.-set. 2021 Epub 30-Set-2021
Original article
Diazotrophy of rhizobia associated with rice plants cv. INCA LP-5 and INCA LP-7
1Instituto Nacional de Ciencias Agrícolas (INCA), carretera San José-Tapaste, km 3½, Gaveta Postal 1, San José de las Lajas, Mayabeque, Cuba. CP 32 700
2Centro de Ciencias Genómicas, Universidad Nacional Autónoma de México. Av. Universidad s/n, Col. Chamilpa, Cuernavaca, Morelos, México. CP 62 210
The rhizobia have been studied, fundamentally, for their capacity to carry out the biological nitrogen fixation, an attribute that at present has also been verified in its interaction with non-legume plants. The aim of this research was to evaluate the capacity to fix nitrogen of rhizobia strains, from the rhizosphere rice plants cultivars INCA LP-5 and INCA LP-7, cultivated in intensive monoculture. The research was carried out in 2018, at the National Institute of Agricultural Sciences from Cuba and at the Center for Genomic Sciences in Mexico. The diazotrophy of three Rhizobium strains was determined by three methods. The amplification of nifH gene, for which three sets of primers and different alignment times and temperatures were used in the PCR technique; through the growth of strains in two semi-solid nitrogen-free media and through inoculation tests with siratro and soy plants, under controlled conditions. The nifH gene was not amplified from any of three rhizobia strains; however, they grew in nitrogen-free media and formed effective nodules on siratro and soy roots. The strain Rhizobium sp. Rpr11 produced the highest number of nodules in both legumes roots, 30 days after inoculation. This research is a first approach to understand the capacity to nitrogen fixation of rhizobia strains associated with Cuban rice cultivars under intensive monoculture.
Key words: nitrogen fixation; grass; symbiosis; Rhizobium
INTRODUCTION
Biological Nitrogen Fixation (BNF) is a very important biological process since it constitutes the only way where the largest nitrogen source on the planet (N2 (g)) is reduced to ammonia, a chemical state that can be assimilated by plants 1. This process is only carried out by a group of prokaryotes (bacteria and archeas), called diazotrophs 2.
Rhizobia are Plant Growth Promoting Bacteria (PGPB) that make the greatest contribution to BNF in the terrestrial planet area. Studies on the symbiotic association of these bacteria with leguminous plants date back at least 125 years and have shown that this interaction contributes around 600 kg N ha-1 year-1 (3,4. The rhizosphere and endosphere of some economically important grasses such as corn (Zea mays L.) and rice (Oryza sativa L.), have also been described as habitats for these bacteria, which is why could to extend to grasses the knowledge and uses of BNF that is known in legumes 5-7.
There are different molecular, physiological and ecological methods that allow classifying a PGPB as diazotrophic. The nifH gene amplification 8, bacterial strains growth in semi-solid nitrogen-free media 9, acetylene reduction assay 10, bacteria inoculation trials in standard legume plants 11) and isotopic techniques with N15 (12 are some examples. In legume plants inoculated with these nitrogen-fixing bacteria, the glutamine synthetase activity in nodules and ureides concentration in nodules and leaves can also be determined 13.
Several studies aboard the hypothesis that phytostimulation is the main mechanism used by rhizobia to promote rice growth and not BNF 14. There is little evidence that shows the presence of nitrogen-fixing rhizobia in ecosystems where legumes have not been present as crops preceding grasses. Studies are lacking in Cuba to determine the capacity to fix nitrogen of rhizobia strains that are naturally associated with economically important grasses such as rice. Therefore, the aim of this research was to evaluate the capacity to fix nitrogen of rhizobia strains from the rhizosphere rice plants cultivars INCA LP-5 and INCA LP-7, cultivated in intensive monoculture.
MATERIALS AND METHODS
The research was carried out in 2018, at the National Institute of Agricultural Sciences (INCA) from Cuba and at the Center of Genomic Sciences from the National Autonomous University of Mexico.
Biological material
Oligonucleotides
Three pairs of degenerate oligonucleotides were used for nifH gene amplification (Table 1). These primers were designed taking into account sequences of the nifH gene of 150 proteobacteria, from nodules of different legumes 15.
Table 1 Oligonucleotides used in the nifH gene amplification three strains of rhizobia
Code | Denomination | Sequence of Nitrogenous Bases (NB) | Number NB |
---|---|---|---|
2087 | nifH_AIF | GTGCTCGGCGACGTGGTRTGCGG | 23 |
2088 | nifH_AIR | ACGATGTTGTCGCGTGGCACGAARTGRATGAG | 32 |
7807 | nifH_UN_FW | CGGGCGTCGGNTGYGCNGG | 19 |
7808 | nifH_UN_RV | CAAAGCCACCGCANACNACRTCNCC | 25 |
180 | KAD | TGCGAYCCSAARGCBGACTC | 20 |
181 | GEM | ATSGCCATCATYTCRCCGGA | 20 |
Bacterial strains
Three rhizobia strains belonging to the strains collection of the Microbiology Laboratory of Plant Physiology and Biochemistry Department from INCA were used. Two strains: Rhizobium sp. Rpr11 (Rpr11) and Rhizobium sp. Rpd16 (Rpd16)from the rhizosphere rice plants´ cultivar INCA LP-5 and Rhizobium sp. 5P1 (5P1) strain from the rhizosphere of cultivar INCA LP-7 16.Both cultivars were established in Gleysol Nodular Ferruginous Petroferric soil from Los Palacios, Pinar del Río, Cuba. When the bacteria isolation was carrying out, this soil is characterized by its low natural fertility, due to its exploitation with intensive rice monoculture for 50 years 17. In addition, two strains were used as positive reference controls: Rhizobium etli CFN42 (CFN42) to detect the nifH gene 18 and Bradyrhizobium elkanii ICA 8001 (ICA 8001) strain in a growth test in nitrogen-free media 19.
Inocula of five rhizobia strains were obtained from roast inoculation in Erlenmeyer flasks (100 mL) with 10 mL of Tryptone-Yeast Extract (TY) medium 20. The Erlenmeyers were kept at 150 rpm and 30 °C, 16 h. Inocula purity was monitored by Gram stain. The cell concentration was determined by the serial dilutions method and cultivation in Petri dishes with solid TY medium; which were incubated 48 h at 30 ºC. The rhizobia concentration was adjusted to 5 x 109 CFU mL-1.
Legume seeds, disinfection and germination
Siratro (Macroptillium atropurpureum) and soybean (Glycine max L.) seeds were used in inoculation trials under controlled conditions. The seeds were superficially disinfected and pre-germinated. The siratro seeds were kept in ethanol (70 %), 5 min they were washed with distilled water and subjected to a treatment with concentrated sulfuric acid, 10 min. They were immersed in sodium hypochlorite solution (25 %), 15 min and they were washed 10 times with sterile distilled water. For the disinfection of soybeans seeds, they were immersed in 70 % ethanol (70 %), 5 min and subsequently in 25 % (v/v) sodium hypochlorite solution (25 %), 15 min. Finally, they were washed 10 times with sterile distilled water. All seeds were placed on plates with water agar (0.75 %) (m/v) and incubated at 30 °C in the dark. The siratro seeds were kept 24 h while the soybeans were 72 h.
Detection of nifH genes in Rhizobium strains
The genomic DNA was extracted from rhizobia strains Rpr11, Rpd16, 5P1 and CFN42, using UltraClean® Microbial DNA Isolation Kit (MOBIO Laboratories, Inc). To verify the extraction success, 5 µL of the extract was analyzed by agarose gel electrophoresis 1.5 % (m/v) in TAE 1X. The time and voltage of electrophoretic run, as well as the molecular weight marker used, were previously described 15.
The nifH gene amplification was carried out by PCR from the genomic DNA extracts. Three pairs of degenerate oligonucleotides (Table 1) were used as primers (3 µL, 0.01 mM) per PCR reaction. The remaining components of PCR mix were: 10X Taq reaction buffer, 5 µL; 25 mM MgCl, 7 µL; Dimethyl sulfoxide (DMSO) 99.5 %, 1 µL; 10 mM dNTPs, 1 µL; Taq polymerase 1 U µL-1, 1 µL; Bacterial DNA 30 ng µL-1, 1.0 µL and 34 µL ultra-pure water; in a final volume of 50 μL per PCR reaction.
The conditions used to perform the PCR were: 1 cycle at 94 ºC, 3 min; 30 cycles with an alignment temperature gradient from 62 ºC to 56 ºC, 15 sec; 72 ºC extension for 1 min and a cycle at 72 ºC, 10 min. The PCR product was analyzed by 1.5 % (m/v) agarose gel electrophoresis in 1X TAE. The time and voltage of electrophoretic run, as well as the molecular weight marker used, were described in previous investigation 15.
Growth of Rhizobium strains in semi-solid nitrogen-free media
Cell suspensions were prepared from Rpr11, Rpd16 and 5P1 strains inoculants. For this, 1 mL of inoculants was centrifuged at 10,000 rpm, 10 min and supernatant was discarded. The pellet was resuspended in 1 mL of sterile sodium chloride solution (0.9 % (m/v)). This procedure was repeated twice to obtain a cell suspension devoid of TY medium components.
Flasks containing 10 mL of semi-solid nitrogen-free Rennie and JMV media inoculated (21 were with 100 μL of bacterial suspensions. Inoculation was performed with a micropipette by releasing the cell suspension from the medium bottom to the surface. Flasks inoculated with 100 μL of sterile sodium chloride solution (0.9 % (m/v)) were used as control and flasks inoculated with same volume of cell suspension of ICA8001 strain were the positive control. The flasks were incubated for five days at 30 ºC and the bacterial growth was determined by the presence of translucent halos on the surface and inside the medium. The change of JMV medium color (green to yellow) was interpreted as organic acid production 21. The test was carried out twice and five flask were used for each bacterial strains.
Nodulation of leguminous plants inoculated with Rhizobium strains
The formation of effective nodules in BNF of the Rpr11, Rpd16 and 5P1 strains, inoculation tests were carried out in siratro and soy plants. Siratro seedlings, with roots approximately 1-2 cm long, were placed in flasks (200 mL) with 50 mL of Norris and Date semi-solid medium 22, and one seedling per flask was placed. The same way, soybean seedlings were placed in test tubes (diameter 2 cm, length 20 cm) with 20 mL of same medium.
Siratro and soybean seedlings were inoculated with 0.5 and 1.0 mL of rhizobi ionocula, respectively. The both legumes seedlings, which were inoculated with sterile LM medium, were used as negative control. The investigation was carried out twice with a Completely Randomized design. Seven plants were used per treatment in siratro and nine for soy.
The plants grew under controlled conditions with a photoperiod of 12 h light/12 h darkness, at a day/night temperature of 26/22 ºC and relative humidity of 70 %. Five weeks after inoculation it was determined: number of nodules in main root; total nodulation; total nodule effectiveness in BNF and dry weight of total nodule. The nodule effectiveness was determined by visual method. The nodules were dissected with stainless steel scalpel blades, detecting or not the presence of a reddish coloration inside the nodules, characteristic of the leghemoglobin protein 23.
Statistical analysis
The data from inoculation tests of siratro and soybean plants were subjected to normality test (Bartlett's test) and variance homogeneity (Kormogorov-Smirnov test). Before the statistical processing, the data were transformed by √x, when x is number of nodules,. Simple classification analysis of variance was applied. The Tukey (P<0.05) mean comparison test was used to discriminate differences between the means. The Statgraphic Plus version 5.0 program was used for the statistical processing of the data.
RESULTS AND DISCUSSION
Most of the works about identification of rhizobia associated with grasses have been carried out in experimental areas subjected to crop rotation techniques with legume plants 24,25. According to these, legumes increase nitrogen-fixing rhizobia populations and the grass is benefiting for nitrogen that remains in the soil from BNF. It has been verified that the nitrogen contribution to rice from some rhizobia did not come from BNF; since the soil where the clover (Trifolium alexandrinum) was cultivated as predecessor crop 14.
In the present investigation, molecular and physiological methods were used to determine the nitrogen fixation capacity of three Rhizobium strains from rice rhizosphere grown in intensive monoculture. It was possible to extract the genomic DNA of rhizobia strains which was visualized as bands in the agarose gel (Figure 1). However, nifH amplification analysis showed that this gene was not amplified with none of three primer pairs that were used. A band (300-400 bp) corresponding to nifH gene amplification of reference strain CFN42 was observed in agarose gel (Figure 2).
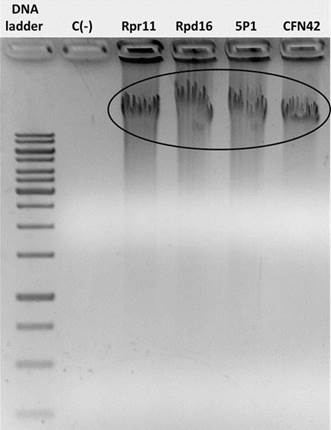
Figure 1 Agarose gel electrophoresis (1.5 %) (m/V) of DNA extracts from the three rhizobia isolated from the rice cultivars INCA LP-5 e INCA LP-7 and reference strain R. etli CFN42; C(-), negative control
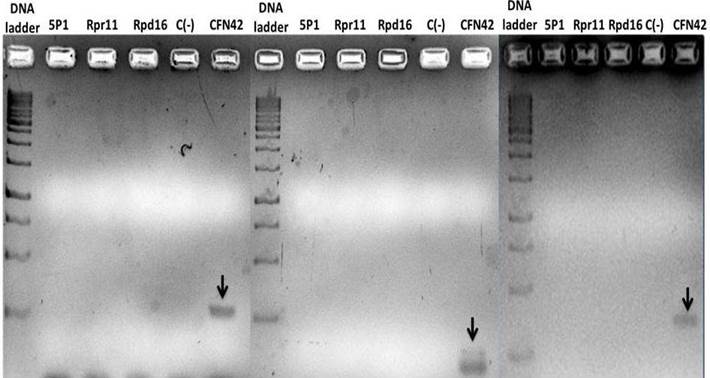
Figure 2 Agarose gel electrophoresis (1.5 %) (m / V) of PCR-amplified nifH gene fragments of three rhizobia isolated from the rice cultivars INCA LP-5 e INCA LP-7 and reference strain R. etli CFN42
Similar results, regarding nifH gene amplification were obtained in previous investigations with Rhizobium strains from rice plants rhizosphere 26. The nifH gene amplification is not only performed to detect the presence of nitrogenase complex in bacteria, since also to perform phylogeny studies with nitrogen-fixing microorganisms 2.
It is known that genes such as nifH need to be amplified with oligonucleotides that contain high degeneracy levels and with adequate temperatures in PCR, which allow hybridization between primers and the corresponding region of bacterial DNA 8. Therefore, degenerate oligonucleotides and a considerable alignment temperature gradient were employed. However, its amplification was not achieved in the Rhizobium strains Rpr11, Rpd16 and 5P1.
Another aspect to consider is the primer sets sequences. Universal and group-specific PCR primers have been developed in order to amplify various sequences of nifHgene. However, most of them only amplify 35 % of sequences from alpha, beta and gamma proteobacteria. The universal primers less than 50 % detect known sequences of this gene, due to the particularly notable variation in the coverage of these primers. Even, previous studies showed that the universal PolF/PolR primers only cover 25% of nifH diversity 8.
The lack of specificity of some nifH gene primers have amplified DNA sequences from Escherichia coli strains, bacteria that until now have not been described as diazotrophic. Primer dimerization, hairpin formation, guanine and cytosine content and primer thermodynamics; are factors that affect the primers specificity and coverage 8. Thus could think that the PCR amplification technique and the lack primers specificity and coverage would explain the non-detection of nifH gene in the Rhizobium isolates studied here, regardless they were designed from nifH gene sequences from different diazotrophic bacteria. The non-detection of nifH gene by the method used here does not necessarily mean that the strains lack the ability to carry out FBN. It would be necessary to use other primer sets that could be designed from sequences from rice associated diazotrophic bacteria.
In this research, the three Rhizobium strains ability to perform BNF was evaluated too, from the physiological point of view. The bacteria growth was showed in two nitrogen-free culture media (JMV and Rennie), after five days of incubation. A change JMV medium color was not observed. Bacillus, Paenibacillus and Azospirillum strains; with a high frequency of appearance in corn and rice rhizosphere; also grow in these culture media 27,28.
Recent studies have demonstrated that rhizobia from grasses grow in nitrogen-free media. Bradyrhizobium and Rhizobium undicola from sorghum (Sorghum bicolor (L.) Moench) and rice, respectively grew in different nitrogen-free media (Rennie and JNFb) 29,30. The diazotrophy of these strains was confirmed by ARA technique. Nitrogen-free media are used in multiple studies in order to select diazotrophic microorganisms and thus discard a large number of culturable microorganisms that are obtained during the isolation process 9.
An inoculation tests were carried out on siratro and soy plants to evaluate Rhizobium strains ability to perform BNF. The results showed effective nodule formation in both legumes inoculated with bacterial strains from rice plants rhizosphere (Figure 3, Table 2).
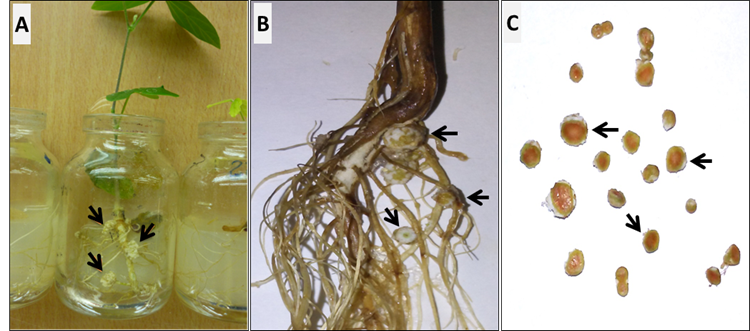
Figure 3 Presence of nodules in siratro (A) and soy (B) roots inoculated with one of the rhizobia isolates
Table 2 Inoculation effect of Rpd16, Rpr11 and 5P1 strains on the root nodulation of siratro and soy plants
Treatments | Nnmr | Tn | TnEf | DWTn |
---|---|---|---|---|
Negative Control | 0,00 b | 0,00 b | 0,00 b | 0,00 b |
Rpd16 | 0,00 b | 0,28 b | 0,29 b | 0,0001 b |
Rpr11 | 1,28 a | 5,85 a | 4,81 a | 0,024 a |
SE x | 0,21* | 0,17* | 0,19* | 0,0002* |
Control | 0,00 b | 0,00 c | 0,00 c | 0,000 c |
Rpd16 | 0,53 ab | 3,13 b | 2,46 b | 0,006 b |
Rpr11 | 0,35 b | 3,78 a | 3,36 a | 0,011 a |
5P1 | 1,27 a | 2,78 b | 2,34 b | 0,006 b |
SE x | 0,22* | 0,14* | 0,20* | 0,001* |
The results correspond to the first experiment repetition due to its similarity with those obtained in the second repetition
Nnmr, number of nodules in the main root; Tn, total nodulation ; TnEf, total nodule effectiveness; DWTn, dry weight of total nodules. Control, plants inoculated with sterile LM medium. Mean with the same letters in the same column do not differ significantly (Tukey α = 0.05 and n = 7 (siratro), n = 9 (soy))
The results showed that the inoculation of siratro seedlings with Rpr11 strain produced the highest values in the nodulation. Nodules were observed in root plants treated with the Rpd16 strain, however there were no significant differences with the control treatment. Siratro is a forage legume that has been established as a model plant to evaluate the infection capacity and nodulation effectiveness of rhizobia isolates under controlled conditions, since responds as a host to wide spectrum of rhizobia strains from different habitats 31.
Regardless of siratro versatility as to nodulate with different rhizobia genera, the plant and bacterium specificity to establish symbiosis has been discussed previously 32,33. Soy, another of legumes used here is generally associated with species of genus Bradyrhizobium34. However, recent research shows that other genera such as Rhizobium, Ensifer, Mesorhizobim and Sinorhizobium also form nodules on their roots 35. The inoculation of 5P1 strain significantly increased nodule number in soy plant main root; whereas, the use of Rpr11 strain produced the highest values in the total nodulation, total nodule effectiveness andand dry weight of total nodule.
The siratro and soy plants inoculated with three Rhizobium strains have root nodules, with a reddish coloration inside (Figure 3C). The brown, red or pink color is characteristic of leghemoglobin, a protein that indicates the effectiveness of the BNF effectiveness 23. This protein has a protoheme-like prosthetic group that binds reversibly with oxygen, which diffuses to central zone of the nodules and transports it from the plasma membrane of infected cells to symbiosome membrane. This mechanism allow establishing oxygen concentrations in nodules to keep the Rhizobium alive and protect nitrogenase enzyme from high oxygen concentrations, and promote BNF 36.
The results of this research are apparently contradictory. The molecular method did not allow nifH gene amplification. However, the bacteria growth in nitrogen-free semi-solid media and nodulation tests in legume plants show that these bacteria fix nitrogen.
The use of more sophisticated molecular methods in taxonomic studies of bacteria allowed reformulating traditional concepts to try to characterize, classify and name of rhizobia strains. Gram-negative bacteria, such as rhizobia, fix nitrogen and form nodules in legumes root (4, which have not been found in the rest of prokaryotes. However, recent studies using total DNA sequencing indicate that both attributes are not necessarily inherent properties of rhizobia 37. These investigations conclude that genus Bradyrhizobium strains have a great diversity in their nif (fixing nitrogen) and nod (nodule formation) genes. This study show that some of these Bradyrhizobium strains have both genes groups, others neither of them and other strains only nif genes; regardless of site from which they were isolated (soil or legume nodules). Therefore, in the study of rhizobia strains, focused on form nodules and fix nitrogen ability, the genetic peculiarities previously described would have to be taken into account.
The ability to fix nitrogen in rhizobia has to consider the possibility of do it, not only in a symbiotic context with legumes, since also in other forms of life forms of these bacteria (free life, associative and endophytic) 37. The three strains studied in this research are examples of rhizobia strains, isolated from the rhizosphere of two economically important rice cultivars (INCA LP-5 and INCA LP-7), that form effective nodules in siratro and soy plants. It would be necessary to corroborate the capacity of these strains to fix nitrogen by methods with greater sensitivity and specificity such as ARA and N15 technique.
Find rhizobia from rice with potential to fix nitrogen in areas under intensive monoculture and, soil with low fertility, provides an important practical value to these bacterial strains. The use of Bioproducts based on these strains could be part of integrated crop management, with less use of mineral fertilizers. This technology acquires special relevance in the context of ecologically and economically sustainable agriculture, especially in crops with high demand and large cultivated areas such as rice. The rice yield is increased each year with a irrational use of mineral fertilizer which negatively affect ecosystems 5.
CONCLUSIONS
Rhizobium strains, associated with rice plants cultivars INCA LP-5 and INCA LP-7 grown in monoculture, seem fix nitrogen, despite not showing nifH gene amplification. The presence of this gene is a strictly necessary condition for nitrogen fixation, however some environmental conditions are also necessary for enhance the process. Therefore, is a necessary modifying existing techniques and even to implement new ones to evaluate the nitrogen-fixing capacity of rhizobia associated with non-legume plants.
This research is a first approach to understand the nitrogen fixation of rhizospheric rhizobia from rice cultivated in in intensive monoculture.
BIBLIOGRAFÍA
1. Saiz E, Sgouridis F, Drijfhout FP, Ullah S. Biological nitrogen fixation in peatlands: Comparison between acetylene reduction assay and 15N2 assimilation methods. Soil Biology and Biochemistry. 2019;131:157-65. [ Links ]
2. Nishihara A, Matsuura K, Tank M, McGlynn SE, Thiel V, Haruta S. Nitrogenase activity in thermophilic chemolithoautotrophic bacteria in the phylum Aquificae isolated under nitrogen-fixing conditions from Nakabusa hot springs. Microbes and environments. 2018;33(4):394-401. [ Links ]
3. Chanway CP, Anand R, Yang H. Nitrogen fixation outside and inside plant tissues. In: Advances in biology and ecology of nitrogen fixation. InTechOpen; 2014. p. 1-21. [ Links ]
4. Sahgal M, Jaggi V. Rhizobia: Culture Collections, Identification, and Methods of Preservation. In: Microbial Resource Conservation. Springer; 2018. p. 175-97. [ Links ]
5. Korshunov VV, Leshchinskaya AF. Mineral fertilizers: in addition to the private control government regulation is required. Russian Journal of Industrial Economics. 2017;10(1):44-52. [ Links ]
6. Zhao J-J, Zhang J, Sun L, Zhang R-J, Zhang C-W, Yin H-Q, et al. Rhizobium oryziradicis sp. nov., isolated from rice roots. International journal of systematic and evolutionary microbiology. 2017;67(4):963-8. [ Links ]
7. Pérez-Pérez R, Oudot M, Serrano L, Hernández I, Nápoles M, Sosa D, et al. Rhizospheric rhizobia identification in maize Zea mays L. plants. Agronomía Colombiana. 2019;37(3):255-62. [ Links ]
8. Gaby JC, Buckley DH. The use of degenerate primers in qPCR analysis of functional genes can cause dramatic quantification bias as revealed by investigation of nifH primer performance. Microbial ecology. 2017;74(3):701-8. [ Links ]
9. Xu J, Kloepper JW, Huang P, McInroy JA, Hu CH. Isolation and characterization of N2-fixing bacteria from giant reed and switchgrass for plant growth promotion and nutrient uptake. Journal of basic microbiology. 2018;58(5):459-71. [ Links ]
10. Fulweiler RW, Heiss EM, Rogener MK, Newell SE, LeCleir GR, Kortebein SM, et al. Examining the impact of acetylene on N-fixation and the active sediment microbial community. Frontiers in Microbiology. 2015;6:418. [ Links ]
11. Howieson JG, Dilworth MJ. Working with rhizobia. Primera. Australia: Australian centre for international agricultural research Canberra; 2016. 314 p. [ Links ]
12. Roley SS, Xue C, Hamilton SK, Tiedje JM, Robertson GP. Isotopic evidence for episodic nitrogen fixation in switchgrass (Panicum virgatum L.). Soil Biology and Biochemistry. 2019;129:90-8. [ Links ]
13. Garg N, Saroy K. Interactive effects of polyamines and arbuscular mycorrhiza in modulating plant biomass, N2 fixation, ureide, and trehalose metabolism in Cajanus cajan (L.) Mill sp. genotypes under nickel stress. Environmental Science and Pollution Research. 2020;27(3):3043-64. [ Links ]
14. Yanni YG, Rizk RY, Abd El-Fattah FK, Squartini A, Corich V, Giacomini A, et al. The beneficial plant growth-promoting association of Rhizobium leguminosarum bv. trifolii with rice roots. Functional Plant Biology. 2001;28(9):845-70. [ Links ]
15. Mora Y, Díaz R, Vargas-Lagunas C, Peralta H, Guerrero G, Aguilar A, et al. Nitrogen-fixing rhizobial strains isolated from common bean seeds: phylogeny, physiology, and genome analysis. Applied and environmental microbiology. 2014;80(18):5644-54. [ Links ]
16. Hernández Forte I, Nápoles García MC. Rizobios residentes en la rizosfera de plantas de arroz (Oryza sativa L.) cultivar INCA LP-5. Cultivos Tropicales. 2017;38(1):39-49. [ Links ]
17. Pozo-Galves C, Cabrera-Alonso JR, Márquez-Reina E, Hernández-Hernández O, Ruiz-Sanchez M, Domínguez-Palacio D. Características y clasificación de suelos Gley Nodular Ferruginoso bajo cultivo intensivo de arroz en Los Palacios. Cultivos Tropicales. 2017;38(4):58-64. [ Links ]
18. González V, Santamaría RI, Bustos P, Hernández-González I, Medrano-Soto A, Moreno-Hagelsieb G, et al. The partitioned Rhizobium etli genome: genetic and metabolic redundancy in seven interacting replicons. Proceedings of the National Academy of Sciences. 2006;103(10):3834-9. [ Links ]
19. Nápoles MC, Martínez J, Costales D, Morales B, Martínez E, Rogel MA. Avances en la reidentificación de la cepa ICA 8001 (Bradyrhizobium japonicum) como perteneciente a Bradyrhizobium elkanii. Revista Cubana de Ciencias Biológicas. 2011;20(1-2). [ Links ]
20. Josey DP, Beynon JL, Johnston AWB, Beringer JE. Strain identification in Rhizobium using intrinsic antibiotic resistance. Journal of Applied Bacteriology. 1979;46(2):343-50. [ Links ]
21. Baldani JI, Reis VM, Videira SS, Boddey LH, Baldani VLD. The art of isolating nitrogen-fixing bacteria from non-leguminous plants using N-free semi-solid media: a practical guide for microbiologists. Plant and soil. 2014;384(1):413-31. [ Links ]
22. Norris DO. Legume bacteriology. In: Tropical pasture research: principles and methods. Commonwealth Agricultural Bureaux; 1976. p. 134-74. [ Links ]
23. Kanu SA, Dakora FD. Symbiotic functioning, structural adaptation, and subcellular organization of root nodules from Psoralea pinnata (L.) plants grown naturally under wetland and upland conditions in the Cape Fynbos of South Africa. Protoplasma. 2017;254(1):137-45. [ Links ]
24. Chaintreuil C, Giraud E, Prin Y, Lorquin J, Bâ A, Gillis M, et al. Photosynthetic bradyrhizobia are natural endophytes of the African wild rice Oryza breviligulata. Applied and environmental microbiology. 2000;66(12):5437-47. [ Links ]
25. Matiru VN, Dakora FD. Potential use of rhizobial bacteria as promoters of plant growth for increased yield in landraces of African cereal crops. African Journal of Biotechnology. 2004;3(1):1-7. [ Links ]
26. Banik A, Mukhopadhaya SK, Dangar TK. Characterization of N2-fixing plant growth promoting endophytic and epiphytic bacterial community of Indian cultivated and wild rice (Oryza spp.) genotypes. Planta. 2016;243(3):799-812. [ Links ]
27. Breedt G, Labuschagne N, Coutinho TA. Seed treatment with selected plant growth-promoting rhizobacteria increases maize yield in the field. Annals of Applied Biology. 2017;171(2):229-36. [ Links ]
28. Ayubb N, Cerra A, Chamorro L, Pérez A. Resistencia a cadmio (Cd) de bacterias endófitas y bacterias rizosféricas aisladas a partir de Oriza sativa en Colombia. Revista Colombiana de Ciencia Animal-RECIA. 2017;9(2):281-93. [ Links ]
29. Singh MK, Singh SK, Singh DP. Novel bacterium Rhizobium undicola isolated from the upland cultivated rice Oryza sativa L. promoting plant growth. Journal of Pharmacognosy and Phytochemistry. 2018;7(3):2437-44. [ Links ]
30. Hara S, Morikawa T, Wasai S, Kasahara Y, Koshiba T, Yamazaki K, et al. Identification of nitrogen-fixing Bradyrhizobium associated with roots of field-grown sorghum by metagenome and proteome analyses. Frontiers in microbiology. 2019;10:407. [ Links ]
31. Lima AS, Nóbrega RSA, Barberi A, da Silva K, Ferreira DF, de Souza Moreira FM. Nitrogen-fixing bacteria communities occurring in soils under different uses in the Western Amazon Region as indicated by nodulation of siratro (Macroptilium atropurpureum). Plant and Soil. 2009;319(1):127-45. [ Links ]
32. Mbengue MD, Hervé C, Debellé F. Nod factor signaling in symbiotic nodulation. In: Advances in Botanical Research. Elsevier; 2020. p. 1-39. [ Links ]
33. Liu C-W, Murray JD. The role of flavonoids in nodulation host-range specificity: an update. Plants. 2016;5(3):33. [ Links ]
34. Wang ET, Tian CF, Chen WF, Young JPW, Chen WX. Ecology and Evolution of Rhizobia. Springer; 2019. [ Links ]
35. Chen WH, Yang SH, Li ZH, Zhang XX, Sui XH, Wang ET, et al. Ensifer shofinae sp. nov., a novel rhizobial species isolated from root nodules of soybean (Glycine max). Systematic and applied microbiology. 2017;40(3):144-9. [ Links ]
36. Tsujimoto R, Kotani H, Yokomizo K, Yamakawa H, Nonaka A, Fujita Y. Functional expression of an oxygen-labile nitrogenase in an oxygenic photosynthetic organism. Scientific reports. 2018;8(1):1-10. [ Links ]
37. Avontuur JR, Palmer M, Beukes CW, Chan WY, Coetzee MP, Blom J, et al. Genome-informed Bradyrhizobium taxonomy: where to from here? Systematic and applied microbiology. 2019;42(4):427-39. [ Links ]
Received: March 15, 2021; Accepted: March 15, 2021