INTRODUCTION
In tropical areas, silage production is a viable alternative for maintaining animal production and minimizing the effects of availability of grasses for ruminants due to their seasonality. Cenchrus purpureus is among the most promising forages of this region for silage production, with a production that ranges between 15 and 43 t of dry matter (DM) ha-1 year-1 (García et al. 2014 and Monteiro et al. 2016). In Cuba, it is the most used forage species after sugar cane due to its high biomass production, good leaf proportion, rusticity and adaptation to a great diversity of soils and adverse climate conditions (García et al. 2014). There are many studies on silages based on C. purpureus, including clones obtained at the Instituto de Ciencia Animal (ICA) like Cuba OM-22 (Castaño and Villa 2017) and Cuba CT-169 (Morales et al. 2016 and Rodríguez et al. 2017).
Production of mixed silages, including protein shrubs, allows the nutritional value of silage to be improved, since tropical grasses have a low nutritional quality, even without being subjected to anaerobic fermentation process for their conservation. Among the protein shrubs, Moringa oleifera is recognized for its high nutritional quality, high protein content and good production of green biomass (Nouman et al 2014). However, the inclusion of high levels of crude protein (CP) could negatively influence on silage quality, because the higher the protein content in fresh forage, the greater will be its buffer capacity or resistance to pH change during the fermentation process (Otero and Esperance 1994). Therefore, under production conditions, it is recommended to include only between 20 and 40% of the shrub in the silages (Rodríguez et al. 2017).
In addition, forages of tropical grasses generally have low contents of soluble carbohydrates (Ferreira et al. 2015), which are fundamental substrates for lactic acid bacteria that guarantee an adequate conservation of plant material. Therefore, the use of energy and microbial additives can help ensure a quality silage for its use in ruminant feed. Sweet potato tuber (Ipomoea batatas) is considered a good source of energy because it has high contents of starch (more than 20 %) and sugars (5-6 %), which generally constitute more than 80% of its DM and are generally higher than those of cassava (Manihot esculenta, Crantz) and maize (Zea mays L.) (Jusuf and Ginting 2017). On the other hand, Vitafert is a biological product, developed in the ICA, which is considered as an activator of fermentation because it stimulates the production of organic acids and lowers pH (Elías and Herrera 2011).
The combination of chemical composition analysis with the estimation of chemical indicators and fermentation kinetics parameters is a good research tool to predict the nutritional value of a food (Mtui et al. 2009). The objective of this study was to evaluate the effect of the inclusion of the biological product Vitafert and sweet potato tuber on the nutritional value of mixed silages of C. purpureus cv. CUBA CT-169 and M. oleifera for ruminants, by means of the analysis of chemical composition and in vitro fermentation of ensiled products.
MATERIALS AND METHODS
Plant material. C. purpureus cv. Cuba CT-169 and M. oleifera (moringa) forage plants, with 90 and 60 days of age, respectively, were collected in the experimental areas of the ICA, in typical red ferralitic soil, of rapid desiccation and uniform profile (Hernández et al. 2015). It is located between 22º 58 N and 82º 02 W and 80 m above sea level. Grass and tree materials were obtained by manual cut. Both materials were freshly carried, chopped in a forage mill until they reached a particle size of 20-30 mm and dried in the sun one day to increase their DM up to 25-30 %.
Silage production. A 70:30 mixture of CT-169 and moringa was prepared, which constituted the fibrous and protein basis of the silages to be evaluated. This was considered as control treatment. As an amylaceous source and energy additive, sweet potato tuber (Ipomoea batatas) was used in four levels of inclusion (0, 5, 10 and 15 % of the total mixture). Sweet potatoes of small and medium size were used, without cleaning but without adhered soil, which were cut lengthwise into pieces and then into thin sheets (± 1.0 mm wide and a diameter smaller than 20 mm).
The biological product Vitafert (1 %) was used as a microbial additive to the silage, which was obtained by fermenting a mixture of final molasses from sugar cane, soybeans, maize, urea, magnesium sulfate, mineral formulas and yogurt as microbial inoculum (Elías and Herrera 2011). For its production, a 250 L capacity stainless steel fermenter was used, with a central piece to homogenize the mixture and an automatic regulator to control the stirring and resting time at 120 and 20 minutes, respectively (Bustamante 2014).
The production of microsilos was carried out in PVC tubes (24 cm x 10 cm), with capacity for 450 g of fresh forage (Gutiérrez et al. 2015). The core of CT-169 and moringa was mixed according to the treatment with the corresponding level of sweet potato and then the Vitafert was sprayed to the obtained mixtures. Treatments were introduced into microsilos and the material was compacted with a tamping tool. Finally, the microsilos were hermetically sealed and kept for 64 days in a protected and dry place. Six microsilos were prepared per treatment. Formulations of evaluated silages were:
{CT -169 + Moringa} (100 %) (Control silage)
{CT-169 + Moringa} (100 %) +Vitafert (1 %) (silage+Vitafert)
{CT-169 + Moringa} (95 %) +Vitafert (1 %) + sweet potato (5 %) (silage+Vitafert+5 %sweet potato)
{CT-169 + Moringa} (90 %) +Vitafert (1 %) + sweet potato (10 %) (silage+Vitafert+10 %sweet potato)
{CT-169 + Moringa} (85 %) +Vitafert (1 %) + sweet potato (15%) (silage+Vitafert+15%sweet potato)
At the end of the silage process, when microsilos were opened, a sample of 10 g of each was taken, 90 mL of distilled water was added and it was mixed in an orbital shaker at 250 rpm for 15 minutes at 20 ºC. Then, the mixture was filtered through gauze and samples were taken from the filtrate for analysis of short chain fatty acids (SCFA) and NH3.
Additionally, approximately 200 g were taken from each microsilo. Samples were dried for 72 h, in forced air oven, with regulated temperature (60 °C). Then, they were milled in a hammer mill until they reached a particle size of 1 mm. Later, half of the dried material from each microsilo was individually stored in sealed nylon bags until the chemical composition analysis was conducted. The rest of the dried material was homogeneously mixed by treatment and the obtained pool was also stored in sealed nylon bags until its use in in vitro evaluations.
In vitro experimental procedure. The in vitro technique of gas production in glass bottles, described by Theodorou et al. (1994), was applied. An amount of 1.0 g of DM from each treatment was incubated in 100 mL bottles, in culture medium (Menke and Steingass 1988) and an inoculum of ruminal microorganisms, in proportion of 0.20 of total incubation volume (80 mL), was also incubated.
The ruminal content of two Siboney de Cuba cows, cannulated in rumen, was used as inoculum. These cows were fed ad libitum with forage grasses and with free access to water and mineral salts. The ruminal content of each animal was collected before offering food in the morning and kept in closed thermos to reach the laboratory, where it was filtered through several layers of gauze and the two inocula were mixed in equal proportions. During the process, temperature of the inocula was 39 ± 1ºC, and the conditions of anaerobiosis by continuous flow of CO2 were maintained. Bottles were sealed and incubated in a water bath at a controlled temperature (39 °C). That moment was taken as the zero hour of the incubation.
Gas production was measured by means of an HD8804 manometer, coupled to a TP804 pressure gauge (DELTA OHM, Italy). After each measurement, the gas was released to equalize the external and internal pressures of the bottles. The volume of gas was estimated from the pressure data using a pre-established linear regression equation (Rodríguez et al. 2013): Gas (mL) = (pressure [103 Pa] 4.95)/2.5858) (n = 132; R2 = 0.9821)
The volume of gas was expressed per gram of incubated (OMinc) organic matter (OM). Two in vitro tests were performed. In the first of the tests, gas production was measured at 3, 6, 9, 12, 16, 20, 24, 30, 48, 72 and 96 hours of incubation to estimate kinetic parameters of fermentation of the evaluated silages, by using the single-phase Gompertz model:
Where:
Y |
is the gas production at time t (mL g-1 MOinc) |
A |
is the gas production potential (asymptote when t = ∞; mL g-1 OMinc). |
B |
is the relative gas production rate |
C |
is a constant factor of microbial efficiency (h-1) |
t |
is the incubation time (h) |
In addition, the time at which the maximum gas production speed (TVmax) was reached was estimated from the second derivative of the Gompertz model, evaluated at zero (inflection point of the sigmoidal model). Maximum gas production speed (Vmax, mL g-1 OMinc h-1) was also estimated, when replacing TVmax in the first derivative of the model (Rodríguez et al. 2013).
In the second trial, incubation was carried out until 24 h. At that time, fermentation was stopped and the bottles opened and their content was filtered through nylon bags (45μm pore size) to separate the solid phase from the incubation liquid. Samples were taken from the filtrate and preserved with a deproteinizing solution (H3PO4 [2 %]) for the analysis of individual SCFA and with HCl (0.2 N) to determine ammonia. The preserved samples were stored at -20 ºC until analysis.
The bags with fermentation residues were dried for 72 h in a forced air oven with a regulated temperature (60 °C). The in vitro degradability of dry matter (IVDDM) was determined by gravimetry, as the difference between DM in the incubated substrate and in the solid residue of fermentation, divided by the incubated DM in each bottle (Blümmel et al. 1997).
The metabolizable energy (ME) and organic matter digestibility (OMD) at 24 h of mixed silages were estimated from the equations proposed by Menke et al. (1979):
Where, PG24h was the volume of gas produced at 24 h (mL • 200 mg-1 incubated DM) and CP is expressed in percent.
Chemical analysis. DM, OM and CP were determined to the samples (AOAC 2016). Neutral detergent fiber (NDF) was obtained by the procedure described by Van Soest et al. (1991) and ammonia analyzes were performed by colorimetry (Chaney and Marbach 1962).
Concentration of individual SCFAs in the preserved samples was determined by gas chromatography, by injecting 0.5 μL, after centrifugation of the vials at 14,200 x g (Centrifuge ECEN-205, MRC Ltd., Hagsvish, Israel) for 8 minutes. A DANI Master GC gas-liquid chromatographer (DANI Instruments S.p.A, Milan, Italy) was used, equipped with a capillary column DN-FFAP (30 m long, internal diameter 0.32 mm, film thickness 0.25 μ) and an FID detector. H2 was used as carrier gas and N2 as auxiliary gas. Maximum temperature of the injector and the detector was set at 200 and 250 °C, respectively. Individual SCFAs determined in the case of mixed silage were expressed as percent of the DM, while the individual SCFAs obtained in the gas production tests were expressed in mmole L-1. Total SCFA (tSCFA) were also obtained by the algebraic sum of determined individual SCFA.
Experimental design. In order to evaluate indicators of chemical composition and of the fermentative process of mixed silages, a completely randomized experimental design was applied, considering silages as treatments (5) and each microsilo as an experimental unit (6).
In the case of the 96-h in vitro experiment, in order to facilitate the analysis of in vitro accumulated gas production, fermentation was divided a priori into two phases. The first phase (initial phase) included five sampling times between the start and 24 hours of incubation (6, 9, 12, 16 and 24 h) and the second phase (final phase) was considered from that moment until the end of incubation, and, in this case, four sampling times were analyzed (30, 48, 72 and 96 h).
As in vitro accumulated gas production is repeatedly measured during the time in the same experimental unit, the fulfillment of theoretical assumptions of normality for the analysis of variance was analyzed with the use of Shapiro-Wilk (Shapiro and Wilk 1965) test and Pearson correlation analysis. As the normality assumption was not fulfilled, the Mixed Generalized Linear Model was used, through the utilization of GLIMMIX of SAS procedure. Treatments, sampling times and interaction treatment per time were considered as fixed effects, the intercept as random effect and the bottle as subject. In order to know distribution for data fit, Normal, Poisson, Log normal and Gamma distributions were tested, being this last the one with the best fit and Log was the bond function. To select the best fitting model, the variance-covariance structures Toeplitz (Toep), Variance Component (CV), Composite Symmetry (CS), Autoregressive of Order 1 (AR [1]) and Unstructured (UN) were tested. To select the variance matrix of best fit to the data, the information criteria [Akaike (AIC), corrected Akaike (AICC) and Bayesian (BIC)] were used, for which the smallest value was considered. The structure that met this requirement was the Toep. Means were compared through the fixed range test (Kramer 1956). For data analysis, the statistical package SAS (2013), version 9.3, was used.
In the second in vitro test, the indicators determined at the end of the 24 hour of incubation (NH3, individual and total SCFA, ME, OMD and IVDDM) were analyzed according to a random block design. Treatments consisted of mixed silages (5), blocks or replicas were the weeks in which incubations were carried out (2), and bottles were considered as repetitions (4). When differences (P <0.05) were found, means of the treatments were compared by multiple rank test of Duncan (1955). Pearson correlation index was used to determine the linear relationship between indicators measured or estimated in the study. The statistical package InfoStat (Di Rienzo et al. 2012) was used for these analyzes and mathematical modeling.
RESULTS AND DISCUSSION
Chemical composition and fermentative indicators of silage process. In none of the open microsilos, the presence of fungi or oxygen was observed, so the storage and manufacturing process allowed an adequate fermentation and the way of sealing was successful. All treatments classified as good quality silage according to their organoleptic characteristics (color, smell and texture).
Table 1 shows the chemical composition of the mixed silages and chemical indicators of fermentation that occurred during the conservation process. No differences were observed in DM, CP and NDF content (P> 0.05). High contents of NDF indicate an appreciable disappearance of soluble material during the anaerobic fermentation process. On the other hand, OM was higher than 85 % in all treatments and only differences were observed for this indicator between control silage and the treatment with 10 % sweet potato (P <0.05).
Low protein content obtained in the designed silage formulations, lower than the indicators reported for traditional silages based on sorghum (9 %) and maize (8%) forages (Ensminger et al. 1990) is noteworthy. This was because moringa, used as main protein source, has high levels of CP (Rodríguez et al. 2014). However, in the present study, it was included in a low proportion in silage mixtures, so its contribution in nitrogen was limited, to which the low protein content of the rest of the components was added. Rodríguez et al. (2017) observed that mixed silages of CT-169 and moringa, in similar mixtures to those used in this study (80:20 and 60:40), had similar DM values, lower in OM (78 %) and NDF (58%), but higher in CP (18 %). Nevertheless, these authors evaluated younger forages than those used in this study, which would explain the lower fiber content and higher protein content.
It could not be appreciated that the inclusion of Vitafert would improve the CP content of silages as observed by Gutiérrez et al. (2014), when evaluating the effect of this microbial additive in mixed silages of Tithonia diversifolia, C. purpureus cv. Cuba CT-169, although these authors evaluated higher inclusion levels of this microbial additive (4.5, 6 and 8 %).
Regarding the fermentative indicators of the evaluated silages, it was observed that the inclusion of the microbial additive and the energy additive increased the acetic production compared to the control treatment (P <0.0001), obtaining the highest increase with the treatment with 5 % of sweet potato tuber inclusion. Regarding this organic acid, acetic acid levels are not so important when classifying the quality of silages since there are several types of lactobacilli that produce it and good quality silages are common, which are obtained from forages poor in proteins, and contain large amounts of this SCFA (Chalacán and Valencia 1999).
On the other hand, no effect of the treatments was observed in the production of propionic acid (P>0.05). The performance of propionic acid did not coincide with that reported by Rêgo et al. (2010), who appreciated that the inclusion of achiote (Bixa orellana) by-products to silages of C. purpureus increased the levels of this SCFA.
Regarding the production of butyric acid, all the treatments with additives reduced the production of this organic acid, with respect to the control (P <0.0001), although the best effect corresponded to silage with Vitafert. The presence of butyric acid in the ensiled material is related to the presence of bacteria of the Clostridium genus, with significant losses in the quality of the silage and the consequent decrease in palatability and voluntary intake (Wilkinson 1983). In the present study, only the silage with Vitafert as an additive showed values lower than the 0.1 % of DM, considered as the maximum accepted value for a silage of excellent quality (Roth and Undersander 1995). Butyric concentrations under this value are a key indicator that a desirable fermentation occurred, a result of low activity of Clostridium bacteria (Roth and Undersander 1995). In the case of the treatment where only the microbial additive was used, this could have a faster decrease of pH and favor lactic acid bacteria growth. On the other hand, the inclusion of sweet potato also contributed to reduce the levels of this SCFA compared to the control silage, although without reaching the value of 0.1 % DM. This effect could be due to the contribution of easily fermented carbohydrates that serve as a substrate for lactic acid bacteria. In any case, other authors consider that silages with concentrations of butyric lower than 0.5 % DM can also be considered as good quality silages (Kung and Shaver 2001).
Simultaneously, there were differences in the NH3 content among treatments, but only the silage with Vitafert and 15 % of sweet potato reduced the levels of this compound with respect to the control silage (P <0.001). In mixed silages of C. purpureus and cocoa meal, Teixeira et al. (2008) observed ammonia values similar to those obtained in the present study (3.9 %).
A problem when using C. purpureus as forage is that its high humidity can affect a high decomposition of its proteins (Viana et al. 2013). Since ammonia is a product of fermentation, especially of clostridial fermentations, the observed levels are satisfactory because they were less than 10-12%, established limit for classifying a silage as good quality McDonald et al. 1991. This confirms that the studied silages were obtained by desirable fermentations, although in the case of control silage, these results did not coincide with the previously analyzed butyric acid levels. These low ammonia values may have been caused in part to the previous pre-drying process to which forages were subjected previous to be ensiled.
Gas production and kinetic parameters of fermentation of the evaluated silages. For the initial phase of the fermentation, it was observed that there was interaction between treatments and sampling times (P<0.0001). Table 2 shows the results of this interaction. In the first three analyzed schedules, silage with Vitafert produced more gas than control silage, but did not show differences regarding the rest of the treatments, except for the two hours that it was lower than gas production of the treatment with 15 % of sweet potato. However, in the following two times (16 and 24 h), there was no difference between control silage and the rest of the treatments, except at 16 h that this treatment produced less gas than silage with 10 % of sweet potato (P <0.001).
Table 2 Effect of interaction among treatments and times of in vitro accumulated gas production of in the initial phase of fermentation (original means appear between brackets)
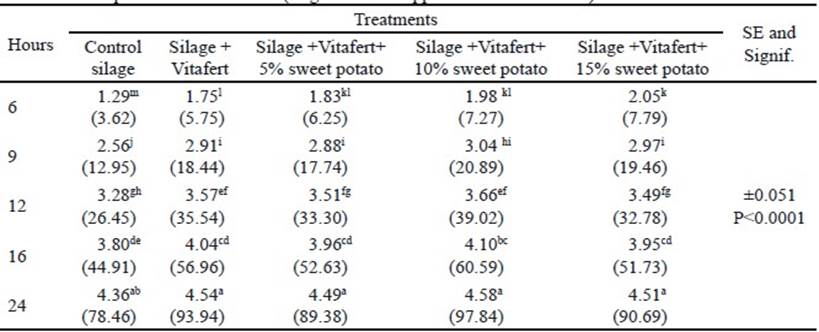
a,b,c,d,e,f,g,h,i,j,k,l,m Different letters indicate significant differences for P<0.05
In the final phase of fermentation, there was no interaction between treatments and sampling times (P>0.05). The individual effects of treatments and sampling times on the in vitro accumulated gas production are shown in tables 3 and 4, respectively. Regarding treatments, all improved gas production compared to control silage (P<0.0001), although there was no difference between silage with Vitafert and silage that also included 5% of sweet potato. The highest gas production corresponded to the silage with the highest tuber proportion in its composition. For their part, times differed among themselves, increasing gas production by increasing sampling hours (P <0.0001).
Table 3 Effect of treatments on in vitro accumulated gas production in the final phase of fermentation (original means appear in brackets)

a,b,c,d Different letters indicate significant differences for P<0.05
Table 4 Effect of times on in vitro accumulated gas production in the final phase of fermentation (original means appear in brackets)

a,b,c,d Different letters indicate significant differences for P<0.05
Table 5 shows the estimated kinetic parameters of the in vitro accumulated gas production profile of the evaluated silages. A tendency to increase gas production potential (parameter A) was observed by including Vitafert and increasing the levels of sweet potato, which may be related to the availability of growth promoting compounds and soluble carbohydrates, respectively. Regarding the use of sweet potato in silages, there are references that confirm a high in vitro digestibility (92.0%) of sweet potato (Backer et al. 1980 and Ruiz et al. 1981). In addition, Rendon et al. (2013) reported that the integral silage of sweet potato (tuber and foliage) showed ruminal degradability of 66.8% when incubated for 72 h, similar values to maize silage.
Table 5 Kinetic parameters according to Gompertz model of in vitro fermentation of the evaluated mixed silages
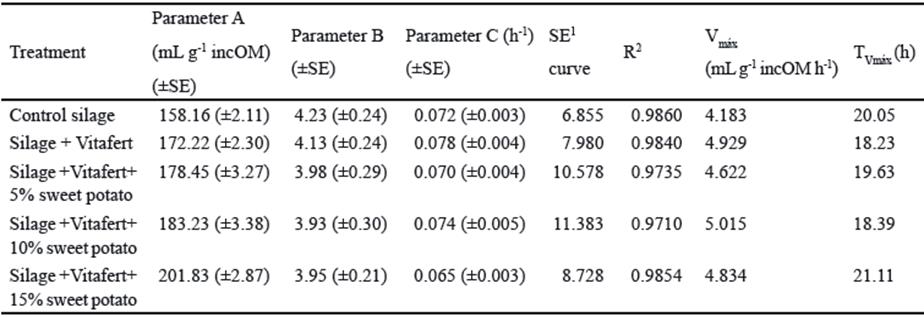
SE of parameters were significant at P˂ 0.0001; 1 SE of the curve
The same trend, but negative, was appreciated for the relative rate of gas production (parameter B). However, it was not appreciated that the Vitafert affected parameter C or sweet potato inclusion level in the silage mixture showed a defined trend on this parameter. On the other hand, the inclusion of additives tended to improve Vmax values although only silage with Vitafert and silage with Vitafert and 10% of sweet potato tended to decrease TVmax. In the present study, all treatments reached their Vmax between 18 and 21 h. When comparing these results with those obtained by Rodríguez et al. (2017), it is appreciated that the obtained Vmax were higher than those reported by these authors, although longer incubation times were reached. Differences between both studies, despite using the same forages, could be given by the evaluated cut ages and their effects on the chemical composition of silages and on the partition of in vitro fermented nutrients towards gas production or microbial synthesis.
Figures 1 and 2 show gas production and gas production speed profiles of the evaluated mixed silages, respectively. Gas production curve of silage treatment with 15 % of sweet potato tended to be higher than the rest of the treatments, which was previously associated to better availability of soluble carbohydrates, provided by the tuber. On the other hand, it was observed that silage with Vitafert and Vitafert with 5 and 10 % of sweet potato, had a similar performance of their fermentation profiles, intermediate between control silage and silage with 15 % of sweet potato.
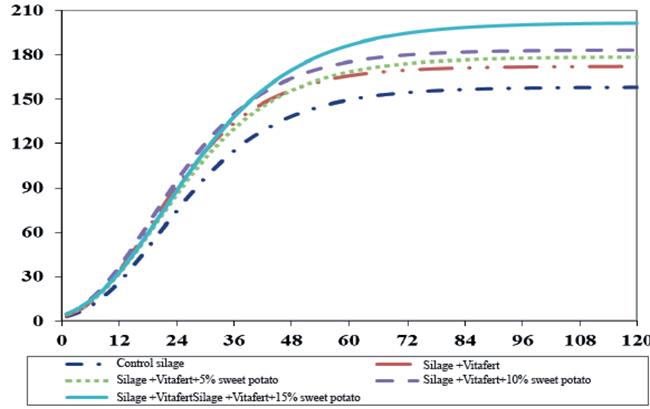
Fig. 1 Profile of in vitro accumulated gas production (Y axis, mL g-1 incOM) in the time (X axis,h) of evaluated mixed silages, estimated from the parameters obtained by Gompertz model
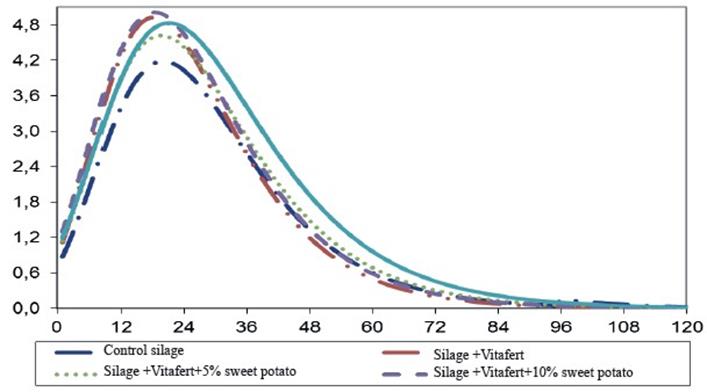
Fig. 2 Profile of in vitro accumulated gas production speed (Y axis, mL g-1 incOM h-1) in the time (X axis, h) of evaluated mixed silages, estimated from the parameters obtained by Gompertz model
Indicators of fermentation up to 24 hours of evaluated mixed silages. Table 6 shows indicators of in vitro fermentation of the evaluated silages. The inclusion of Vitafert and sweet potato decreased the content of ammonia, although the lowest value of NH3 belonged to the treatment of silage with Vitafert (P< 0.001). When correlating levels of NH3 at 24 h of incubation with CP content of the evaluated silages, there was a high linear correlation between these two variables (r= 0.8314). It is important to state that concentrations of NH3 in all treatments surpassed the value of 5 mg 100 mL-1, considered as minimum for a good functioning of ruminal ecosystem (Satter and Styler 1974).
Tabla 6 Indicators of in vitro fermentation of the evaluated mixed silages
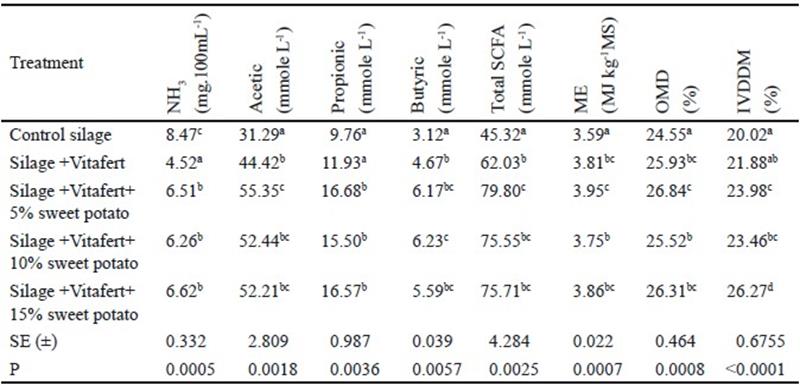
a,b,c,d Different letters indicate significant differences for P<0.05
Likewise, the inclusion of Vitafert and sweet potato increased the production of acetic and butyric acids, as well as the estimated production of total SCFAs, ME and OMD (P< 0.01), but only treatments with sweet potato improved the production of propionic acid (P< 0.01).
When determining DM degradability by gravimetry, there were no differences between control silage and silage with Vitafert, but there was an increase of IVDDM with the inclusion of sweet potato in the composition of silages (P< 0.0001), obtaining the best degradability with 15 % of sweet potato inclusion.
When correlating values of estimated OMD and the IVDDM determined by gravimetry, there was a high correlation between both variables (r= 0.7551). This is an element to be taken into consideration because variable OMD may be used for estimating, using gas production and CP content, the effect of treatments on degradability and, this way, substituting gravimetric determinations, which sometimes are difficult and lead to losses of non-fermented materials, and subsequently, overestimate IVDDM.
Conclusions
The inclusion of Vitafert and sweet potato, at the evaluated concentrations, had no significant influence on chemical composition of the evaluated mixed silages. However, both additives improved the fermentative characteristics of silages with the reduction of the levels of butyric acid and the increase of propionic acid. Likewise, their use improved the nutritional value of ensiled products because they optimized the performance of kinetic parameters of in vitro fermentation and increased gas production, individual and total SCFAs, IVDDM and estimations of ME and OMD, with a positive effect of sweet potato inclusion level on some of these indicators.
Out of these results, it is recommended to evaluate superior inclusion levels of sweet potato to increase availability of non-structural carbohydrates in the ensiled product. In addition, using the low levels of CP and NH3, it should be evaluated the increase of protein content in the mixtures to be ensiled from the use of superior proportions of the protein shrub or small amounts of urea. Finally, the use of Vitafert is a viable option for small and medium farmers, but it should be useful to evaluate pure strains of microorganisms with potential as additives for silages, which will constitute a feasible option for developing products for large-scale farmers, who consider that rusticity of Vitafert production is not viable.