Introduction
Infectious diseases represent a continuous and increasing threat to human health and welfare. They are the major causes for vast morbidity and mortality in the world, although developing countries are carrying the major part of the problem (Tariq et al., 2019). The number of infections caused by new, reemerging, or antibiotic resistant pathogens is growing daily, and the increased proportion of patients with immunodeficiency has resulted in an increase of severe and invasive infections (WHO, 2016). Hence, there is an urgent need to find alternative antimicrobial agents for the treatment of resistant pathogenic microorganisms.
Among the alternatives studied, many essential oils have been used in folk medicine throughout the world, and their medicinal properties have been investigated (Saeidnejad & Rajaei, 2015; Ambrosio et al., 2017; Tariq et al., 2019). Eucalyptus globulus Labill. (Myrtaceae) is the principal source of eucalyptus leaf oil in the world and exhibit antibacterial, antifungal, analgesic and anti-inflammatory properties and have also been widely used in pharmaceutical, food and cosmetics products (Giles et al., 2010; Damjanović-Vratnica et al., 2011; Mulyaningsih et al., 2011; Elaissi et al., 2011; Tyagi & Malik, 2011; Harkat-Madouri et al., 2015; Luís et al., 2016). The main component of the essential oil is the monoterpene oxide 1,8-cineole, also known as eucalyptol, being the amount of this compound between 44 and 84% and it is known to possess significant antimicrobial activity (Ishnava et al., 2013; Goldbeck et al., 2014).
Generally, it is recognized that plant secondary metabolites do not remain stabilized like other qualitative and quantitative traits. The influencing factors responsible for those fluctuation can be divided into genetic, ontogenic, morphogenetic and environmental factors. All these four principal factors are important in the production or accumulation of plant secondary metabolites and are the reasons of the fluctuations in chemical composition and biological activities (Verma & Shukla, 2015).
Several species of bacteria are pathogenic and cause infectious diseases, such as the Gram-positive bacterium Staphylococcus aureus, which is mainly responsible for post-operative wound infection, toxic shock syndrome and food poisoning. Escherichia coli, a Gram-negative bacteria, is present in human intestines and causes urinary tract infection, cholecystitis or septicemia. The Gram-positive bacterium Streptococcus pyogenes cause a variety of diseases such as streptococcal pharyngitis, rheumatic fever, rheumatic heart disease, and scarlet fever (Reddy et al., 2012).
The present study was undertaken (i) to analyze the chemical composition of the leaf essential oil of Eucalyptus glogulus Labill. grown in two regions of heights of the canton Cañar and (ii) to assess their antimicrobial properties on three clinically significant bacterial strains essential oils.
Materials and Methods
Materials
Leaves of eucalyptus were collected in two regions of the canton Cañar in Ecuador: Moyancón (1347 m height above sea level) and parish Chorocópte (3191 m height above sea level), in March 2018. The identity of the plant specimens was confirmed and registered in the herbarium of the National Park of the Catholic University of Cuenca, where a voucher specimen was deposited (EUC-104).
Essential oil isolation
The fresh leaves (100 g) were submitted to hydrodistillation in a Clevenger-type apparatus for two hours. Isolations were made by duplicate. The essential oils were dried over anhydrous sodium sulfate and stored in hermetically sealed dark-glass containers at -4 °C until further analyses.
Gas chromatography-flame ionization detector and gas-chromatography-mass spectrometry
Analyses of the essential oils were performed by gas chromatography with a flame ionization detector (GC-FID) on an HP-6890 instrument gas chromatograph (Hewlett-Packard Co., Palo Alto, CA) equipped with a 30 m × 0.25 mm i.d. × 0.25 mm DB-5ms (J & W Scientific, Folsom, CA, USA). The analyses were conducted under the following conditions for both columns: oven temperature program, 70 °C (2 min), 70-240 °C (4 °C/min) and 240 °C (5 min); carrier gas helium flow rate 1 mL/min; injector and detector temperatures 250 °C, injection volume 0.2 μL and split ratio 20:1.
Essential oils were also analyzed by gas chromatography-mass spectrometry (GC-MS) using a GC-MS QP-2010 Ultra (Shimadzu, Kyoto, Japan) fitted with a 30 m × 0.25 mm i.d. × 0.25 mm BP-5 (SGE Analytical Science Pty. Ltd., Victoria, Australia) column. GC parameters were like GC-FID and interface temperature: 250 °C; MS source temperature: 230 °C; MS quadrupole temperature: 150 °C; ionization energy: 70 eV; mass range: 35-350 m/z. The different components were identified using the retention indices and mass spectra. Retention indices, calculated using linear interpolation relative to retention times of C6-C24 of n-alkanes, were compared with those standards and data from the literature (Adams, 2001). Mass spectra were compared with corresponding reference standard data reported in the literature (Adams, 2001) and mass spectra from NIST 05, Wiley 6, NBS 75 k, and in-house Flavorlib libraries. In many cases, the essential oils were subject to co-chromatography with authentic compounds. The quantification of compounds was performed using relative percentage abundance and normalization method with correction response factors based on grouping the essential oil components by their functional groups (Costa et al., 2008). Percentage data are the mean values of two injections per sample.
Antibacterial assay
The minimal inhibitory concentration (MIC) of the essential oil was determined by broth microdilution methods (Mulyaningsih et al., 2010). Briefly, the samples were pipetted into 96-well microtiter plates in Mueller Hinton broth (Fluka, Switzerland) followed by a twofold serial dilution. An inoculum suspension was added to give a final concentration of 5 × 105 cfu/mL. After incubation at 37 °C for 24 h, MIC was determined as the lowest concentration without bacterial growth. The minimal bactericidal concentration (MBC) was determined by subculturing 3 μL from each well without apparent microbial growth on Columbia 5% sheep blood agar and incubated at 36 °C for 24 h. The lowest concentration without apparent microbial growth was taken as the MBC. The experiments were performed in duplicate and repeated twice. Three bacterial strains were used for the antimicrobial studies: two Gram-positive reference strains: Staphylococus aureus ATCC 25923 and Streptococus pyogenes ATCC 28422 and one clinical isolate Eschericha coli (Gram-negative).
Results and Discussion
The essential oil yields of leaves from Moyancón and Chorocópte regions were 0.17 % and 0.15 % (v/m), respectively. Both essential oils had a spicy aromatic odor. These results were rather small compared to the results reported in the literature where the yield was 1-3% (Giles et al., 2010; Damjanović-Vratnica et al., 2011; Mulyaningsih et al., 2011; Elaissi et al., 2011; Tyagi & Malik, 2011; Ishnava et al., 2013; Goldbeck et al., 2014; Harkat-Madouri et al., 2015). No significant differences were found in the essential oil yields from the two regions of heights of the canton Cañar.
The chemical composition of the leaf oils of E. globulus grown in two regions of heights of the canton Cañar was determined by GLC-MS (Table 1). A total of 99 compounds were unambiguously identified, representing 100% of the total composition. Monoterpenoids were predominantly found in both essential oils.
Table 1 Chemical composition of the essential oils from E. globulus leaves collected in two heights of the canton Cañar
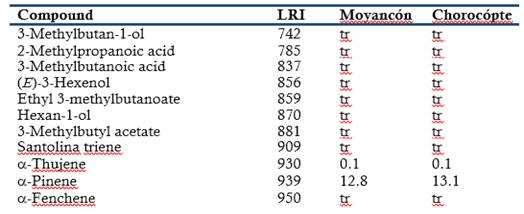
The essential oils contained mainly 1,8-cineole (67.4 and 67.6% in Moyancón and Chorocópte regions, respectively) and α-pinene (12.8 and 13.1%) were the main compounds. The hydrocarbon (E)-caryophyllene was the major sesquiterpene. The leaf oil of E. globulus is well known to be a 1,8-cineole-rich oil (Giles et al., 2010; Damjanović-Vratnica et al., 2011; Mulyaningsih et al., 2011; Elaissi et al., 2011; Tyagi & Malik, 2011; Ishnava et al., 2013; Goldbeck et al., 2014; Harkat-Madouri et al., 2015; Luís et al., 2016). Some differences can occur in composition of essential oils from the same species probably due to genetic variation and different environmental factors, such as climate, harvesting seasons and geographical location (Verma & Shukla, 2015). No significant differences were found in the chemical composition of the essential oils from the two regions of heights of the canton Cañar.
Table 2 Minimum inhibitory concentration (MIC) and minimum bactericidal concentration (MBC) values of the essential oils from E. globulus leaves collected in the canton Cañar
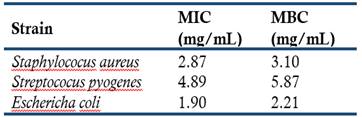
Considering that both essential oils have the same composition, only the oil sample from Moyancón area was evaluated for its antibacterial activity. The MIC and MBC values of the eucalypt essential oil are presented in Table 2 and vary from 1.90 to 5.87 mg/mL. The essential oil showed moderate activities against the evaluated strains because, in general, the MIC and MBC were > 2 mg/mL. Mulyaningsih et al. (2011) reported a MIC value of > 4 mg/mL for E. coli, while Damjanović-Vratnica et al. (2011) found lower MIC and MBC values for S. aureus (0.09 and 0.18 mg/mL, respectively), S. pyogenes (0.09 and 0.09 mg/mL) and E. coli (0.09 and 0.18 mg/mL). On the other hand, Tyagi and Malik (2011) reported MIC and MBC values of 4.5 and 9 mg/mL for E. coli.
Conclusions
The leaf essential oils of Eucalyptus globulus Labill. plants, which grow to 1347 and 3191 m height above sea level in the canton Cañar, Ecuador, had the same chemical composition, which was dominated mainly by 1,8-cineole (>67% of total composition). No significant differences were found between both chemical composition of the essential oils. The essential oil showed moderate antibacterial activity against Staphylococus aureus, Streptococus pyogenes and Eschericha coli.