Meu SciELO
Serviços Personalizados
Journal
Artigo
Indicadores
-
Citado por SciELO
Links relacionados
-
Similares em SciELO
Compartilhar
Cultivos Tropicales
versão impressa ISSN 0258-5936versão On-line ISSN 1819-4087
cultrop vol.42 no.2 La Habana abr.-jun. 2021 Epub 30-Jun-2021
Original article
Chitosan induces defensive responses in soybean plants inoculated with Bradyrhizobium elkanii
1Instituto Nacional de Ciencias Agrícolas (INCA), carretera San José-Tapaste, km 3½, Gaveta Postal 1, San José de las Lajas, Mayabeque, Cuba. CP 32 700
2Fyteko, Allée de la Recherche 4, 1070 Anderlecht, Belgium
Chitosan is a natural polymer that induces numerous biological responses in many plant species, for instance, the induction of enzymatic and metabolic defense responses to biotic stress. In this paper, basal induced resistance enzymes (β 1-3 Glucanase, Chitinase and Phenylalanine-ammonia-lyase) and protein content, were determined in soybean organs, inoculated with Bradyrhizobium and growth in culture media added with different chitosan concentrations. Defensive response induced by the polymer depended on its concentration, the organ of the plant and the moment evaluated. The behavior of enzyme levels was different in soybean leaves and roots and inversely proportional to protein content. Enzymatic values in leaves were higher at 7 days post-treatment than at 21 days, except β 1-3 glucanase activity. However, in the roots, the increases occurred at 21 days, except chitinase activity. Higher concentrations (100, 500 and 1000 mg L-1) of chitosan increased the enzyme levels in roots, mainly at 21 days after inoculation with B. elkanii. Results show the possibility of using chitosaccharides for the induction of defensive responses in soybean against pathogens, without affecting the symbiotic process carried out with Bradyrhizobium elkanii.
Key words: β1-3 glucanase; chitinase; defensive induction; MAMP; PAL
INTRODUCTION
Plants show various defensive reactions when they perceive molecular patterns associated with microorganisms (MAMP) that can be recognized by receptors on the plasma membrane 1-3. Legumes are capable of detecting MAMPs and thus recognizing and discriminating between rhizobia and pathogens to trigger the symbiosis process or the defense response, respectively. The nodulation factors (Nod) produced by the different rhizobia are responsible for initiating the nodule formation process in the roots and, in their basic structure, have chitin oligosaccharides that are recognized inducers of plant defensive responses 3, while other specific compounds derived from rhizobia modulate defensive induction in host plants and benefit symbiotic infection 4-6.
Chitin, a polysaccharide present in the cell wall of fungi, and its derivatives (chitosans and partially N-acetylated chitooligosaccharides) are released by degradation of the fungal cell wall by enzymes β 1-3 glucanases and chitinases. These enzymes are secreted by the plant in response to pathogen attack in the pathogenesis process 7,8. Chitosans have been implicated in the induction of a wide variety of defense-related responses in various plant systems, such as the expression of defensive genes, the induction of phytoalexins and resistance proteins, cell wall lignification, among others 9,10. This induced response in plants depends on numerous factors, such as plant species, the pathogenic microorganism, chitosan physicochemical characteristics and the way in which they are applied 11.
Previous studies in soybean cv INCAsoy-27 demonstrated the negative effect of high chitosan polymer concentrations and oligosaccharides added to the plant culture medium on nodulation response and in vitro growth variables of the seedlings inoculated with Bradyrhizobium elkanii12. The negative effect found in soybean nodulation with the chitosan polymer could be related to the inhibitory action at concentrations of 500 and 1000 mg L-1 on symbiont viability. This affectation could also be related to defensive response stimulation in the seedling by chitosan, which would directly affect the microsymbiont entry to the roots and would compromise the energy balance required for other growth functions in the plant.
Therefore, the hypothesis of this work was to know if the presence of a chitosan polymer in the culture medium of soybean growth inoculated with Bradyrhizobium causes defensive indicator induction of (activity β 1-3 glucanase, chitinase and phenylalanine ammonia- lyase, PAL) on the leaves and roots of the seedlings.
MATERIALS AND METHODS
Chitosan compound
A chitosan polymer with a molecular mass of 130,000 g mol-1 and 12 % deacetylation was obtained from lobster chitin (Panulinus argus) exoskeleton by basic deacetylation (NaOH), which was characterized by some authors 12. Aliquots of a standard chitosan solution dissolved in acetic acid (1 %) were taken, which were added to the Norris & Date plant culture medium 13, up to a final concentration of 0, 10, 50, 100, 500 and 1000 mg L-1.
Soybean seedling growth bioassay
Soybeans from cultivar INCAsoy-27 were previously disinfected with ethanol (70 %) and sodium hypochlorite (0.25 %, v/v) for five minutes, and they were rinsed six times with sterile distilled water before placing them in plates Petri dishes containing agar-water medium (0.75 %) for germination, at 30 °C for three days in the dark. Then, the pregerminated seeds (15-20 mm of emergent root) were placed in test tubes containing 40 mL of semi-solid medium 13 and the different concentrations of the polymer, at the rate of one seed per tube. The roots of the seedlings were inoculated seven days later, discharging with a micropipette 1 mL of inoculum obtained with the ICA 8001 strain of B. elkanii (109 CFU mL-1) in liquid medium of yeast extract-mannitol (YEM). The seedlings were grown in a growth chamber under controlled light/dark conditions for 16/8 hours, at 25 °C and 70 % relative humidity, with artificial light of 1 x 104 moles m-2 s-1 for 35 days. During this time, sterile Norris & Date liquid medium was added weekly to all test tubes to ensure good seedling development. Six seedlings were selected per treatment for the conformation of three homogeneous samples of fresh leaves and roots, for total soluble protein quantification and enzymatic activity determinations, at 7 and 21 days after inoculation with the bacteria (dai). In the roots, the nodules were removed, washed with distilled water and drained on filter paper before their conservation in liquid nitrogen, until plant extraction moment.
Protein extraction in soybean leaves and roots
For the total protein extractions, one gram of the samples of both organs was weighed, which were macerated with liquid nitrogen in a porcelain mortar and homogenized in sodium acetate solution (0.05 mol L-1, pH 5.2)+sodium chloride (1 mol L-1)+EDTA (0.005 mol L-1)+Mercapto-ethanol (0.005 mol L-1), at a rate of 1.5 mL g-1 per sample. The extract was centrifuged at 12,000 g for 15 minutes at 5 °C in a refrigerated centrifuge, its precipitate was discarded and it was centrifuged again for 5 minutes. The supernatant was collected and frozen below 0 °C until the enzymatic determinations were made. Protein quantification was carried out in the supernatant following the Micro Lowry methodology 14. The absorbance was read at 750 nm and 0.5 mg mL-1 of bovine serum albumin (BSA) was used as a standard for the calibration curve with a concentration range from 5 to 100 µg. The protein content was expressed as mg protein g-1 from fresh leaves and roots.
Determination of enzymatic activities
The enzymatic activity β 1-3 glucanase (EC 3.2.1.6) was determined using the methodology described by some authors 14. The assay components were proportionally adjusted to obtain a final volume of 0.5 mL. The reducing sugars released from laminarin (Sigma, 5 mg L-1) by action of extract enzymes (0.025 mL) were quantified. The reaction mixture was incubated for one hour at 50 °C. The total reducing sugars released in the test were determined using the Somogyi colorimetric method 15 and its results were expressed as specific activity (mg of glucosamine-1 min-1 per mg of protein-1), based on the straight line equation of the reducing sugars curve for a glucose standard, at 520 nm.
Chitinase activity (EC 3.2.1.15) was estimated through reducing sugars released by colloidal chitin. The standard assay (0.5 mL) contained enzyme extract (0.03 mL), 0.25 mL of colloidal chitin in 50 mM sodium acetate buffer (pH 5.2), and 0.21 mL of the same buffer. After incubating for four hours at 37 °C under continuous linear shaking (250 rpm), potassium hydroxide (0.01 mL, 5N) was added to the mixture to stop the reaction. The test solution was centrifuged for five minutes (12.000 g) to precipitate the unreacted substrate and determine the content of reducing sugars in the supernatant 14. The calculation of chitinase (mg N-acetyl glucosamine min-1 per mg protein-1) was carried out from the equation of the line of the reducing sugars curve for an N-acetyl glucosamine pattern at 520 nm.
The phenylalanine ammonium-lyase activity (PAL, EC 4.3.1.5) was determined according to the methodology of some authors 14. The assay was incubated for two hours. The trans-cinnamic acid formed by the action of PAL enzymes present in the extract (0.1 mL) was quantified using L-phenylalanine (Sigma, 0.1 mol L-1, pH = 8.8) as substrate. This enzyme was expressed as specific activity (mg of trans-cinnamic acid min-1 per mg of protein-1), after previously determining the equation of the straight line of the trans-cinnamic acid curve at 290 nm.
Statistical analysis
In the experiment, a Completely Randomized Design with 9 repetitions per treatment was used for the indicators: soluble proteins and defensive enzymes of the plant organs (leaves and roots). The data obtained from each indicator were analyzed by Simple Classification ANOVA. The resulting means were compared using the Tukey HSD Test for p <0.05 in the Statgraphics program, version 5.1 (Statistical Graphics Corporation, 2001). Pearson's correlations were performed at the two evaluated moments (7 and 21 ddi) and between organs (leaves and roots) of soybeans, using the resulting means per treatment in all variables.
RESULTS
Figure 1 shows the protein content in leaves and roots during in vitro growth of soybean plants treated with chitosan and inoculated with Bradyrhizobium.
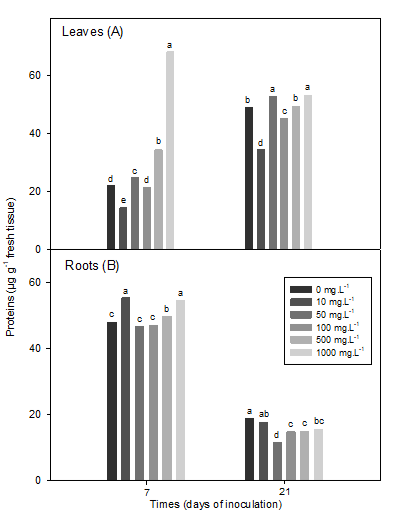
Total soluble proteins in leaves (7 dai (SEx = 0.40 *), 21 dai (SEx = 0.44 *) and roots (7 dai (SEx = 0.40 *), 21 dai (SEx = 0.50 *) of inoculated soybean plants Means with common letters do not differ statistically for p <0.05, according to the Tukey HSD Multiple Range Test
Figure 1 Effect of a chitosan polymer added to the vegetable culture medium on the content of total soluble proteins
The content of total soluble proteins in the leaves was less than 7 dai compared to 21 dai, except for the highest concentration of polymer (1000 mg L-1), which significantly exceeded the rest of the concentrations in this variable, at the 7 dai (Figure 1A). In addition, the concentrations of 50 and 500 mg L-1, with differences between them, accumulated higher content of total proteins with values higher than the inoculated plants (0 mg L-1).
A similar behavior was observed at 21 dai, since the highest values were registered with concentrations of 50 and 1000 mg L-1. At both moments evaluated, the 10 mg L-1 polymer concentration decreased the total protein content (Figure 1A). However, the soluble protein content in roots showed a different response (Figure 1B). The levels decreased significantly in each treatment at 21 dai, except for the lowest polymer concentration (10 mg L-1), compared to the control treatment. In contrast to the protein response in leaves at 7ddi, the concentration of 10 mg L-1 improved protein synthesis in roots, without differences with by 500 mg L-1. The remaining concentrations did not differ from the control treatment (Figure 1B).
Concerning the leaves, the concentrations of 50 to 1000 mg L-1 of the polymer reduced the levels of activity of β 1-3 glucanase until reaching five times less than the control value, at seven days. The response of this enzyme was variable at 21 dai. The 10 and 100 mg L-1 concentrations of chitosan increased the enzymatic activity above the control, while it was reduced by the 500 and 1000 mg L-1 concentrations (Figure 2A).
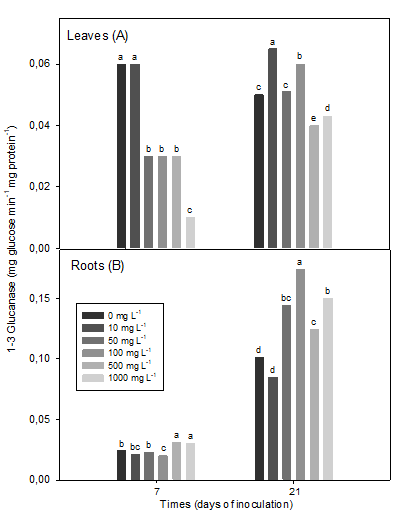
β 1-3 glucanase in leaves (7 dai (SEx = 0.006 *), 21 ddi (SEx = 0.006 *) and roots (7 dai (SEx = 0.007 *), 21 dai (SEx = 0.012 *) of inoculated soybean plants Means with common letters do not differ statistically for p <0.05, according to the Tukey HSD Multiple Range Test
Figure 2 Effect of a chitosan polymer added to the plant culture medium on the β 1-3 glucanase enzyme activity
However, in roots, β 1-3 glucanase activity increased at 7 dai with high concentrations (500 and 1000 mg L-1) as opposed to 100 mg L-1 of the polymer (Figure 2B). On the other hand, at 21 dai, concentrations greater than 50 mg L-1, mainly 100 mg L-1, increased this activity (Figure 2B).
The lower concentration (10 mg L-1) of chitosan reduced the β 1-3 glucanase in roots, but without differences with the control (Figure 2B). Interestingly, the absolute values of the enzymatic activity were almost five times higher at 21 dai than at 7 days after the inoculation of the plants.
At 7 dai, the chitinase activity in leaves decreased with increasing chitosan concentration above 100 mg L-1, while at 21 dai in plants, enzyme activity levels decreased with 1000 mg L-1 one. The rest of the other concentrations did not differ from the control at both evaluated moments (Figure 3A).
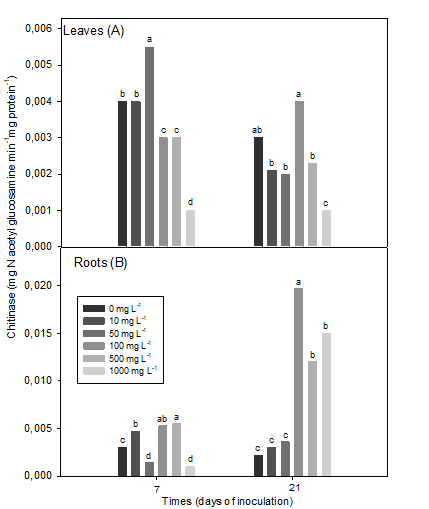
Chitinase in leaves (7 dai (SEx = 0.0002 *), 21 dai (SEx = 0.0002 *) and roots (7 dai (SEx = 0.0002 *), 21 dai (SEx = 0.0007 *) of inoculated soybean plants Means with common letters do not differ statistically for p <0.05, according to the Tukey HSD Multiple Range Test
Figure 3 Effect of a chitosan polymer added to the plant culture medium on chitinase enzyme activity
In contrast, the highest chitinase activity in soybean roots, at 7 ddi, was observed with the concentration of 500 mg L-1 of the polymer, followed by 100 and 10 mg L-1 (Figure 3B).
Chitosan concentrations 50 and 1000 mg L-1 reduced the enzyme in relation to the control. However, at 21 dai, the plants treated with the concentration of 100 mg L-1 of chitosan significantly increased the chitinase activity, followed by the highest concentrations (500 and 1000 mg L-1), which increased the enzymatic activity four to six times more than the remaining concentrations and the control plants (Figure 3B).
The phenylalanine ammonium-lyase (PAL) levels in soybean leaves were higher in the first time of sampling evaluated, than in the second (Figure 4A). The concentrations of 10 and 50 mg L-1 of the polymer increased the PAL enzyme at 7 and 21 dai, respectively.
In leaves, chitosan increased PAL activity up to 75% with a concentration of 10 mg L-1 at 7 dai, compared to the inoculated control. On the other hand, the higher concentrations of the polymer reduced these enzymatic levels in the leaves at 7 dai, while at 21 dai this effect was only observed in plants treated with 1000 mg L-1 of chitosan (Figure 4A).
PAL activity in roots was similar to the response found at 21 dai with β 1-3 glucanase activity (Figure 4B).
The 50 mg L-1 concentration caused the highest levels of PAL activity, followed by the highest concentrations at 7 dai, while 10 mg L-1 and the control showed the lowest levels of activity. However, at 21 dai, the concentrations of 100 and 1000 mg L-1 of chitosan increased this enzyme activity of (Figure 4B).
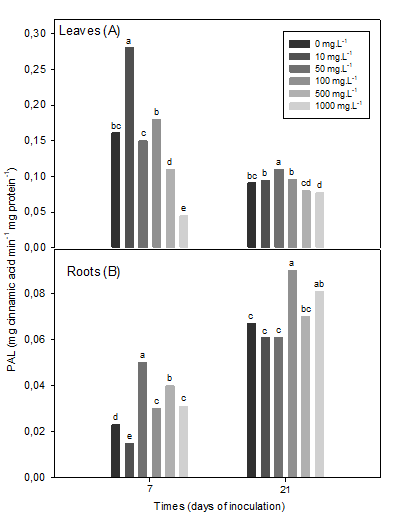
Phenylalanine ammonium lyase (PAL) in leaves (7 dai (SEx = 0.006 *), 21 dai (SEx = 0.003 *) and roots (7 dai (SEx = 0.0006 *), 21 ddi (SEx = 0.003 *) of inoculated soybean plants The means with common letters do not differ statistically for p <0.05, according to the Tukey HSD multiple range test
Figure 4 Effect of a chitosan polymer added to the plant culture medium on the activity of phenylalanine ammonium-lyase (PAL)
The correlation coefficient determinations of the total proteins and the three enzymatic activities, between both plant organs, showed positive and negative correlations, both 7 days and 21 days after inoculation in soybeans (Table 1).
Table 1 Determination of Pearson's correlation coefficients of protein content and enzymatic activities in soybean organs
Variables compared variables | Pearson's correlation coefficients | ||||
---|---|---|---|---|---|
Days | Roots | Leaves | |||
Proteins | β 1-3 Glucanase | PAL | Chitinase | ||
7 | Proteins | -0.603* | ---- | ---- | ---- |
β 1-3 Glucanase | ---- | 0.412 ns | ---- | ---- | |
Chitinase | ---- | ---- | -0.021 ns | ||
PAL | ---- | ---- | 0.769* | ---- | |
21 | Proteins | 0.958* | ---- | ---- | ---- |
β 1-3 Glucanase | ---- | -0.87* | ---- | ---- | |
Chitinase | ---- | ---- | ---- | -0.581* | |
PAL | ---- | ---- | 0.673* | ---- |
(β 1-3 Glucanase, PAL and chitinase) in leaves and roots of soybean plants, at 7 and 21 days after inoculation with B. elkanii
* Level of significance of the coefficient of determination of the Pearson correlation for p <0.05
The application of chitosan in the vegetable culture medium increased the levels of total proteins in both roots and leaves (Table 1). A positive and more significant correlation (R2=92) was found in the concentration levels of the indicator in both organs at 21 dai, than at 7 dai. Similar behavior was found in PAL enzyme activity, where both organs correlated positively and significantly at 7 dai (R2=76) and 21 dai (R2=59) (Table 1). However, β 1-3 glucanase activity was inversely and significantly correlated (R2=76) between both organs, so that increases in activity of this enzyme in leaves correspond to significant reductions in activity in roots at 21 dai , while the correlation at 7 dai was not significant. In turn, chitinase activity increased in roots, but decreased in leaves, so the correlation between organs was negative and of low significance in the two growth moments of the evaluated plants (Table 1).
DISCUSSION
The activities β1-3 glucanase, chitinase and phenylalanine ammonium lyase (PAL) are considered indicators of basal resistance against phytopathogens 16. Likewise, these enzymes have been previously evaluated in plants treated with chitosan 17,18. It is known that chitosan and its derivatives are potent inducers of defensive responses and resistance against pathogens and increase the synthesis of secondary metabolites in plants 8,17. This work shows that chitosan modulated the enzymatic levels of the defensive responses in soybean leaves and roots inoculated with B. elkanii, depending on the plant organ and the moment evaluated. Furthermore, these increases are in the range of levels reported in plants treated with chitosan 18.
In general, at both moments in which the defensive indicators were evaluated, the three highest concentrations caused significant decreases and increases in enzyme levels in leaves and roots, respectively, compared to the control (Figures 2, 33 and 4). Consequently, the enzymatic increases found confirm a defensive induction stimulated by the chitosan polymer in soybean roots, which could make it possible to increase the plant resistance status against possible infections by pathogens.
Among the PR proteins, the enzymes chitinases and β 1-3 glucanases have been the most studied and reported in response to biotic pathogens and elicitors 16,17. The results of this work demonstrated increases in both enzymes´ activity with 10 mg L-1 of chitosan in the leaves, differently in roots, where concentrations between 100 and 1000 mg L-1 stood out, at both times. At 21 days, in the case of β 1-3 glucanase, increases of up to two times were achieved, while in chitinase case, between three and four times in the roots, with respect to the control plants (Figures 2 and 3).
Similarly, the same concentrations that induced the highest enzyme increases in this work have been reported as inducers of chitinase activity in soybean leaves by seed imbibition prior to sowing 19. Other results in this culture showed increases in chitinase activity, depending on the concentration of chitosan used 20.
Some authors demonstrated greater accumulation of chitinase activity in roots grown in solutions that contained a chitosan oligomer or a Nod factor 21; without affecting the nodulation of soybean plants. This suggests that this enzymatic activity stimulation may be due both to a defensive response and to the symbiotic association of rhizobia with the host plant. However, in several legumes species it has been reported that chitinase enzymes hydrolyze Nod factors excreted by bacteria, and therefore, reduce the recognition of these signals by the plant 22,23. This can be supported by the decrease in the number and dry mass of the nodules observed in soybean plants grown in vitro treated with high chitosan concentrations included in the culture medium 12. This is consistent with the increase in β 1-3 glucanase and chitinase activity observed in the present study in plants treated with the highest concentrations of the polymer.
PAL activity in the leaves increased at concentrations of 10 and 50 mg L-1 of the polymer at 21 dai, while with intermediate to high concentrations, enzyme levels decreased at both times of soybean growth (Figure 4A) . However, in the roots at seven days, PAL activity was induced with the highest concentrations, mainly 50 mg L-1, which doubled the control value (Figure 3B). Similar responses were reported in the induction of this activity by chitosan in tobacco roots (Nicotiana tabacum L) and pine (Pinus koraiensis) plants with this concentration 19,24, in addition to finding increases of this enzymatic activity in soybeans and other legumes when other forms of the inducer are applied 18,25-27.
Some compounds formed in the phenylpropanoid pathway, such as flavonoids and isoflavonoids, are involved in the process of symbiotic recognition between rhizobia and host plants 28,29. It is well known that chitosan activates the formation of secondary compounds via phenylpropanoids, reinforcing the resistance in plants to biotic stresses 18,30, but also being able to contribute positively to the aforementioned symbiotic process 31. The increased PAL activity found in soybean roots in this study could contribute to both processes. For example, an increase in flavonoids in soybeans has been reported as a result of the previous chitosan application 8,22) or Nod factors to plants 28,32.
However, defensive response induction in plants against pathogens has been reported to inhibit the symbiotic process in legumes 33. The enzymatic activities in this work were determined in two important moments of the soybean symbiotic process: at 7 dai, when the plant is in full formation of nodules, after the colonization of root hairs by the bacteria and their conversion to bacteroide and at 21 dai, when the nodules are formed and the bacteroides within them are active, exerting an intense nitrogen fixation activity. Furthermore, legumes have been shown to self-regulate the magnitude of symbiosis by inducing defensive responses 4 that limit microsymbiont entry and thus cause the formation of less developed or dysfunctional nodules 33. In this sense, chitosan could induce defense mechanisms in the plant that would directly affect the microsymbiont entry to plant roots.
The results of the work also demonstrated an activation relationship of the variables evaluated in the two organs, depending on the moment evaluated. At 7 dai the proteins were negatively correlated and PAL activity positively, while at 21 dai the four variables correlated between roots and leaves (Table 1).
The protein concentration increased in both organs with the application of chitosan at 1000 mg L-1 and in roots at 21 days when only the seedlings were inoculated. Both behaviors coincide with previous results reported in cowpea plants 34,35.
At 21 days, the higher chitosan concentrations increased the β 1-3 glucanase and chitinase activities in roots, while they were reduced in leaves. Although this behavior may be linked to an energy balance in the plant itself, additional research is necessary to elucidate the biological significance of the observed behaviors. Therefore, it is necessary to evaluate the inducing chitosan effect in other defensive response regulation that could be involved in improving or preventing the symbiotic process of soybeans with Bradyrhizobium, in ex vitro bioassays, beyond the increase in basal plant resistance against pathogens.
CONCLUSIONS
The results of the work showed that chitosan induces protein accumulation and modulates the defensive enzymatic indicators related to plant protection against pathogen attack, depending on the concentration, the plant organ and the soy in vitro growth stage inoculated with Bradyrhizobium.
In the future, eliciting induction of chitosan should be deepened through other forms of application and development moments of inoculated soybeans and under ex vitro conditions.
BIBLIOGRAFÍA
1. Indrasumunar A, Wilde J, Hayashi S, Li D, Gresshoff PM. Functional analysis of duplicated Symbiosis Receptor Kinase (SymRK) genes during nodulation and mycorrhizal infection in soybean (Glycine max). Journal of Plant Physiology. 2015, 176:157-168. [ Links ]
2. Fesel P, Zuccaro A. ß-glucan: Crucial component of the fungal cell wall and elusive MAMP in plants. Fungal Genetics and Biology. 2016, 90:53-60. [ Links ]
3. Wang Q, Liu J, Zhu H. Genetic and Molecular Mechanisms Underlying Symbiotic Speci?city in Legume-Rhizobium Interactions. Front. Plant Sci. 2018, 9(313):1-8. [ Links ]
4. Okazaki S, Tittabutr P, Teulet A, Thouin J, Fardoux J, Chaintreuil C, Gully D, Arrighi JF, Furuta N, Miwa H, Yasuda M, Nouwen N, Teaumroong N, Giraud E. Rhizobium-legume symbiosis in the absence of Nod factors: two possible scenarios with or without the T3SS. The ISME Journal. 2016, 10:64-74. [ Links ]
5. Marczak M, Mazur A, Koper P, Zebracki K, Skorupska A. Synthesis of Rhizobial Exopolysaccharides and Their Importance for Symbiosis with Legume Plants. Genes. 2017, 8(360):1-24. [ Links ]
6. Xiang Q-W, Bai J, Cai J,Huang Q-Y, Wang Y, Liang Y,Zhong Z, Wagner C, Xie Z-P, Staehelin C. NopD of Bradyrhizobium sp. XS1150 Possesses SUMO Protease Activity. Front. Microbiol. 2020,11(386):1-12. [ Links ]
7. Hassan O, Chang T. Chitosan for eco-friendly control of plant disease. Asian J. Plant Pathol. 2017, 11:53-70. [ Links ]
8. Muktar Ahmed KB, Khan MMA, Siddiqui H, Jahan A. Chitosan and its oligosaccharides, a promosing option for sustainable crop production- a review. Carbohydrate Polymers. 2019. [ Links ]
9. Sharif R, Mujtaba M, Ur Rahman M, Shalmani A, Ahmad H, Anwar T, Tianchan D, Wang X. The Multifunctional Role of Chitosan in Horticultural Crops. A Review. Molecules. 2018, 23:872. [ Links ]
10. Locateli BT, Preus da Cruz M, Dalacosta NL, Oligine KF, Bertoldo E, Mazaro SM, Haas J, Potrich M, Favetti CI. Elicitor-induced defense response in soybean plants challenged by Bemisia tabaci. Journal of Agricultural Science. 2019, 11(2):251-262. [ Links ]
11. Orzali L, Corsi B, Forni C, Riccioni L. Chitosan in Agriculture: A New Challenge for Managing Plant Disease. Chapter 2, IN INTECH: Biological Activities and Application of Marine Polysaccharides, 2017; 17-33 p. [ Links ]
12. Costales D, Falcón-Rodríguez AB, Nápoles MC, De Winter J, Gerbaux P, Onderwater RCA, Wattiez R, Cabrera JC. Effect of chitosaccharides in nodulation and growth in vitro of inoculated soybean. American Journal of Plant Science. 2016, 7:1380-1391. [ Links ]
13. Norris DO, Date RA. Legume bacteriology Tropical Pasteur Research. Principles and Methods. C.A.B. Bill. 1976, 51:134-174. [ Links ]
14. Falcón-Rodríguez AB, Costales-Menéndez D, Cabrera JC, Martínez-Téllez MA. Chitosan physic-chemical properties modulate defense responses and resistance in tobacco plants against the Oomycetes Phytophthora nicotianae. Pesticide Biochemical Physiology. 2011, 100(3):221-228. [ Links ]
15. Somogyi M. Notes on sugar determination. J. Biol. Chem. 1952, 195:19-23. [ Links ]
16. Van Loon LC, Pieterse CMJ. Significance of inducible defense-related proteins in infected plants. Annu. Rev. Phytopathology. 2006, 44:135-162. [ Links ]
17. Falcón AB, Costales D, González-Peña D, Nápoles MC. Nuevos productos naturales para la agricultura: las oligosacarinas. Cultivos Tropicales. 2015, 36(1):111-129 [ Links ]
18. Chandra S, Chakraborty N, Dasgupta A, Sarkar J, Panda k, Acharya K. Chitosan nanoparticles: A positive modulator of innate immune responses in plants. Scientific Reports. 2015, 5(15195):1-14. [ Links ]
19. Falcón AB, Días D, Ramírez MA. Hidrólisis enzimática de quitosano. Actividad biológica del polímero y sus hidrolizados. Cultivos Tropicales. 2009, 25(2):81-86. [ Links ]
20. Falcón AB, Cabrera JC, Ortega E, Martínez MA. Concentration and physicochemical properties of chitosan derivatives determine the induction of defense responses in roots and leaves of tobacco (Nicotiana tabacum L.) plants. Am. J. Agric. Biol. Sciences. 2009, 4(3):192-200. [ Links ]
21. Xie Z-P, Staehelin C, Wiemken A, Broughton WJ, Muller J, Boller T. Symbiosis-stimulated chitinase isoenzymes of soybean (Glycine max (L.) Merr.). Journal of Experimental Botany. 1999, 50:327-333. [ Links ]
22. Al-Tawaha AM, Seguin P, Smith DL, Beaulieu C. Biotic elicitors as a means of increasing isoflavone concentration of soybean seeds. Ann. Appl. Biol. 2005; 146:303-310. [ Links ]
23. Jung WJ, Mabood F, Souleimanov A, Park RO, Smith DL. Chitinases produced by Paenibacillus illinoisensis and Bacillus thuringiensis subsp. pakistani degrade Nod factor from Bradyrhizobium japonicum. Microbiological Research. 2008, 163(3):345-349. [ Links ]
24. Liu R, Wang Z-Y, Li T-T, Wang F, An J. The role of chitosan in polyphenols accumulation and induction of defense enzymes in Pinus koraiensis seedlings. CJPE. 2014, 38(7):749-756. [ Links ]
25. Prapagdee B, Kotchadat K, Kumsopa A, Visarathanont N. The role of chitosan in protection of soybean from sudden death syndrome caused by Fusarium solani f. sp. Glycines. Bioresource Technology. 2007, 98(7):1353-1358. [ Links ]
26. Xing K, Zhu X, Peng X, Qin S. Chitosan antimicrobial and eliciting properties for pest control in agriculture: a review. Agronomy for Sustainable Development, Springer Verlag/EDP Sciences/INRA, 2015, 35(2):569-588. [ Links ]
27. Smith DS, Gravel V, Yergeau E. Signaling in the Phytomicrobiome. Published in: Frontiers in Plant Science and Frontiers in Microbiology. 2017, 8(611):1-104. [ Links ]
28. Liu CW, Murray JD. The Role of Flavonoids in Nodulation Host-Range Specificity: An Update. Plants. 2016, 5(3):33. [ Links ]
29. Biala W, Jasínski M. The Phenylpropanoid Case - It Is Transport That Matters. Front. Plant Sci. 2018, 9:1610. [ Links ]
30. Kumar GP, Desai S, Moerschbacher BM, Gueddari NE-E. Seed treatment with chitosan synergizes plant growth promoting ability of Pseudomonas aeruginosa-P17 in sorghum (Sorghum bicolor L.). bioRxiv. 2019;601328. [ Links ]
31. Mathesius U. Flavonoid Functions in Plants and Their Interactions with Other Organisms. Plants. 2018, 7(2):30. [ Links ]
32. Arora NK, Mehnaz S, Balestrini R. Developing improved bioformulations: Future desing and considerations. In: Bioformulations: for sustainable Agriculture. 2016; 236-251 p. [ Links ]
33. Mithofer A. Suppresion of plant defense in rhizobia-legume symbiosis. Trends Plant Sci. 2002, 7:440-444. [ Links ]
34. El-Tanahy AMM, Mahmoud AR, Abde-Mouty MM, Ali AH. Effect of Chitosan Doses and Nitrogen Sources on the Growth, Yield and Seed Quality of Cowpea. Australian Journal of Basic and Applied Sciences. 2012, 6(4):115-121. [ Links ]
35. Viera da Silva L, Braz S, Araujo L, Cerqueira A, Bonifacio A. Coinoculation con Bradyrhizobium and trichoderma alleviates the effects of salt stress in cowpea. Revista Coatinga, Mossoró. 2019, 32:336-344. [ Links ]
Received: November 12, 2019; Accepted: January 27, 2021