Introduction
Chitosan is a cationic polysaccharide composed of β-(1-4)-D-glucosamine and β-(1-4)-N-acetyl-D-glucosamine. It is generally obtained by alkaline deacetylation of chitin, a natural polysaccharide composed of β-(1-4) linked N-acetyl-D-glucosamine units present in the shells of crustaceans, mollusks, the cuticle of insects and the cell walls of fungi.1,2 Chitosan is a biocompatible, biodegradable, nontoxic and possesses other important biological properties such as mucoadhesivity, wound healing capacity, hemostatic and antimicrobial activities.3,4,5,6
Chitosan is soluble in dilute solutions of many organic and inorganic acids, producing highly viscous solutions. It forms chelates with transition and post-transition metal ions and can be processed into gels, films, fibers, microspheres-microcapsules, and micro/nanoparticles. Due to its exceptional physical, chemical and biological properties, chitosan find applications in medicine, pharmacy, cosmetics, agriculture and the food industry.7,8,9) The excellent film forming capacity of chitosan together with its antimicrobial activity has been profited for wound coatings and food preservation.10,11
The wide applicability of chitosan films has incentivized the study of their physical properties, such as water vapor and oxygen permeability, swelling, crystallinity, tensile strength, elongation, glass transition temperature and thermal stability. It has been found that these properties are influenced by the degree of acetylation (DA) and molecular weight of chitosan, the solvent system and the pH of the solution.12 The addition of plasticizer agents, dispersants and compatibilizers can modify these properties, although they can affect the antimicrobial activity of films.13 However, chitosan films are generally prepared from acetic acid solutions and the effect of different acids have only been addressed in few reports. For instance, effect of the solvent (acetic, formic, lactic and propionic) on the mechanical and permeation properties chitosan films was studied by Caner et al.12 Later on, Park et al.14 investigated the effects of acetic, lactic, malic, and citric acid on the mechanical and barrier properties of the films. In a different study, Romanazzi et al.15 studied the effect of chitosan dissolved in different acids (acetic, L-ascorbic, formic, L-glutamic, hydrochloric, lactic, maleic, malic, phosphorous, and succinic acid) on its ability to control postharvest gray mold of table grape. However, to our knowledge only the thermal stability of chitosan films obtained from acetic acid solutions has been reported.
The aim of the present study was to prepare chitosan films using different organic and inorganic acids without plasticizer and to investigate their effect on the thermal properties of the films.
Materials and methods
Materials
Chitosan (MW 2,6 × 105) with degree of deacetylation of 79 % was produced by the Laboratory of Analysis and Development of Biomaterials from Northeast - CERTBIO (Brazil). Acetic acid was obtained from Dinamica Quimica Contemporanea (Brazil), lactic acid (85 %) was purchased from Anidrol (Brazil), citric and ascorbic acid were obtained Sigma Aldrich (USA). Tartaric acid (99 %) was provided by Nuclear (Brazil), maleic acid (99 %) and hydrochloric acid (37 %) by Neon (Brazil) and nitric acid (65 %) by VetecQuimica (Brazil). All other reagents and chemicals were analytical grade.
Preparation of chitosan films
The films were prepared by the solvent evaporation method, by dissolving chitosan in acid solutions, in order to obtain final polymer concentration of 2 % (m/v). Chitosan (2g) was dispersed in water under stirring and then the minimum amount of the selected acid required for dissolution was added. Once chitosan was dissolved, more water was added to achieve a final volume of 100 mL. The solution was centrifuged at 3 500 rpm for 8 minto remove bubbles and any undissolved particles. Then, it was poured into a levelled Petri dish of 100 mm in diameter. The films were dried for 48 h at 50 °C.
The peeled films were stored at ambient conditions until used (nominally 25 °C, 50 - 70 % RH). The films were labelled as follows to indicate the acid used for preparing the solution: CS-Ac (chitosan in acetic acid); CS-Cit (chitosan in citric acid); CS-La (chitosan in lactic acid); CS-Ma (chitosan in maleic acid); CS-Tar (chitosan in tartaric acid); CS-HCl (chitosan in hydrochloric acid) and CS-Nit (chitosan in nitric acid). The original chitosan powder was named as CS.
Thickness of films
Film thickness was measured using a MDC-25SBmicrometer (MITUTOYO CORPORATION, Japan). Samples were tested at 10 random points, and the mean value was calculated.
Infrared spectroscopy
Fourier-transform infrared spectroscopy (FTIR) analysis was performed in a Perkin Elmer spectrometer model Spectrum 400 series (USA). The spectra were determined in the range from 400 to 4 000 cm−1. All the readings were performed at room temperature (25 ºC).
X-ray diffraction
The X-ray diffraction (XRD) analysis was performed with a XRD-7000 diffractometer (SHIMADZU, Japan), with angular scan of 5° < 2θ< 80° in Bragg-Bretano mounting, θ-2θ system, with a step size of 0,02º using a Cu radiation (k = 1,54).
Thermogravimetric analysis
A Perkin Elmer model Pyris 1 (USA) thermogravimetric analyser was used to determine the weight variation of samples upon heating. Approximately10 mg of each sample was placed in an alumina pan with an empty pan used as reference. Samples were heated from 25 to 900 ºC at a heating rate of 10 ºC∙min-1 under an airflow of 20 mL∙min-1. The Universal Analysis 2000 software, version 4.7, from TA Instruments, was used to plot the thermogravimetric (TG) and differential thermogravimetric (DTG) curves. The Origin Pro 8.5 software was used to analyse the curves.
Differential scanning calorimetry
The differential scanning calorimetry (DSC) analysis was performed with a DSC equipment model DSC Q20 (TA Instruments, USA). Approximately 6 mg of sample was placed in an aluminum pan and sealed hermetically with an empty pan of the same type (used as a reference). Measurements were made from 25 to 450 °C at a heating rate of 10 ºC∙min-1 under nitrogen atmosphere with a flow rate of 50 mL∙min-1. The Origin Pro 8.5 software was also used to analyse the curves.
Results and discussion
All films were prepared by casting. The amount of solution poured into the Petri dishes was only the necessary one to achieve a final thickness of approximately100 μm. This quantity was calculated from measurements of the densities of films previously prepared.
The films obtained were transparent and flexible with average thickness ranging from 80 to 110 μm. Films prepared with acetic, lactic and maleic acid were almost colourless. The ones produced with citric and tartaric acid had a slightly yellow appearance and those obtained with nitric and hydrochloric acid were slightly reddish.
Fourier transform infrared (FTIR) spectroscopy
FTIR spectra were obtained in order to investigate the structural difference and the interaction between chitosan and organic and inorganic acids. Figure 1A and figure 1B shows the FTIR spectra of chitosan powder and films prepared from different acid solutions in the wavenumber range of 500-4 000 cm−1.
The spectrum of chitosan powder (CS) is shown in fig. 1a. The broad absorption band in the region 3 500-3 000 cm−1 corresponding to N-H and O-H stretching indicates intermolecular hydrogen bonding of chitosan molecules.16 Also present in the CS spectrum are the distinctive absorption bands at 2 942-2 784 cm-1 (aliphatic C-H stretching), 1 650 cm-1 (Amide I), 1 590 cm-1 (-NH2 bending) and 1 314 cm-1 (Amide III). The CH2 bending and CH3 symmetrical deformations are manifested by the presence of absorption bands at around 1 420 and 1 376 cm−1, respectively. The absorption bands at 1 154 cm-1 (anti-symmetric stretching of the C-O-C bridge), 1 082 and 1 032 cm-1 (skeletal vibrations involving the C-O stretching) are characteristic of its saccharide structure.17,18
The spectra of chitosan films in fig. 1a and b exhibited the wide absorption band at 3 500-3 000 cm−1observed in chitosan powder. Also present in the spectra of all films are the absorption bands in the region 1 180-950 cm-1 characteristic of the polysaccharide. A common feature in all chitosan films is the shift to higher frequencies of the absorption bands at 2 942 - 2 784 cm-1 and the shift to lower frequencies of the bands at 1 650, 1 590, 1 420 and 1 376 cm-1. The change in the characteristic shape of the chitosan spectrum, as well as the peak shifts to a lower frequency range in chitosan films obtained from organic acid solutions showed the same patterns reported by Park and others.19 These shifts may have been caused by different interactions in the matrix, such as interaction of the acid proton with the nitrogen atom of amine group of chitosan 20, inter-molecular rearrangement and variations in the configuration of the main chain.21,22 The differences observed in the peak pattern of chitosan films reflect differences in the intermolecular and intramolecular interactions, which may have their influence on the film properties.
The spectra of CS-La, CS-Cit, CS-Ma and CS-Tar films exhibited a distinctive absorption band at 1 724-1 703 cm-1 not present in the spectrum of the CS-Ac film indicating the presence of an excess of carboxylic groups in these films.23 The absorption band at 862 cm-1 in the CS-Ma film has been assigned to the out of the O=C-C=C-C=O plane C-H deformation from its more stable conformation in maleic acid.24 It is worth noting that the spectra of chitosan films prepared with hydrochloric acid (CS-HCl) and nitric acid (CS-Nit) solutions show very similar absorption bands at 1 625 and 1 526 cm-1 indicating that both strong inorganic acids cause similar interactions with the carbonyl (C=O-NHR) and amine groups of chitosan.
X-ray diffraction study
The X-ray diffractograms (XRD) of chitosan powder and the films prepared from solutions in different acids are shown in fig. 2. The XRD pattern of chitosan powder is characteristic of the hydrated polymorph 25 and exhibited quite sharp and narrow diffraction peaks indicating high crystallinity. The main peaks appear at 2θ = 10,8°, corresponding to the (020)h planes of the hydrated crystalline structure and around 20°, resulting from the contribution of two peaks at 20,16 and 21,8°, related to the reflections of (200)h and (220)h planes of the hydrated polymorph.26 The crystallinity index (CrI) of chitosan powder was estimated from the diffractogram of fig. 2 using the following equation
where I200 is the maximum intensity (at 2θ = 20°) of the reflection (200)h for the hydrated allomorph and Iam is the intensity of the amorphous halo at 2θ = 16°.27 The CrI value obtained for chitosan powder was 68 %.
The XRD of chitosan films show an amorphous diffraction pattern. This is more evident in CS-Cit, CS-La, CS-Ma, and CS-Tar films, which present a broad maximum around 20-22º instead of the characteristic sharp peaks at 2θ = 10,8° and 20° found in the diffractogram of chitosan powder. This is in agreement with literature reports and could be caused by the difficulty of chitosan chains to recover its original close-packed crystalline form when films are casted from the acid solution. It has been pointed out that he intramolecular interactions between the -NH3 + and hydroxyl groups in chitosan limits the molecular movement of the chitosan chain.28 This, combined with the presence of the acid counter-ions might have hindered the formation of inter- and intramolecular hydrogen bonds in chitosan making difficult to attain the closed-packed arrangement required for crystallization.29,30) The possible presence of some acid excess might also contribute to the decreased crystallinity of films.
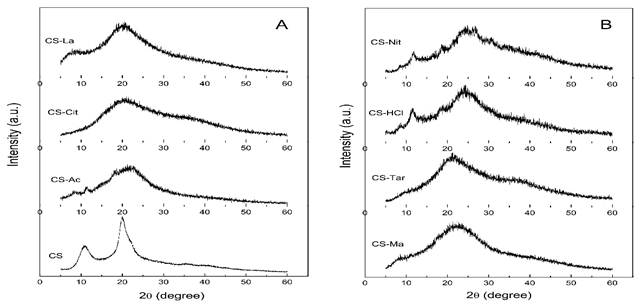
Fig. 2 a), b). X-ray diffraction patterns of chitosan powder (CS) and chitosan films prepared from different organic and inorganic acid solutions.
The diffractograms of CS-Ac films exhibited weak crystalline peaks at 11,8, 16,4, and 22º in 2θ, which was in agreement with literature reports.31,32) The films casted from HCl and HNO3 solutions showed weak crystalline peaks around 8,5, 11,2, 18,6 y 24,5º in 2θ, indicating a disruptive effect on the crystalline structure of chitosan.
Thermal behaviour of chitosan and chitosan films
Thermal analysis was carried in order to study the changes provoked by the acid solvents on the stability of films. The thermal gravimetric (TG) and differential thermal gravimetric (DTA) curves of chitosan powder and the films are shown in fig. 3 and the temperature values of the thermal decomposition stages are listed in table 1, together with their associated weight losses. The differential scanning calorimetry (DSC) curves of CS and chitosan films, in the temperature interval 25 - 450 ºC, are disclosed in fig. 4.
Table 1 Thermal degradation stages and associated weight losses of samples
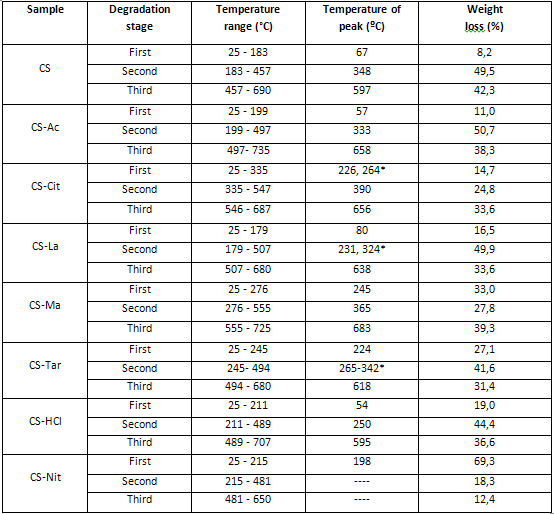
*Two peaks in the Interval
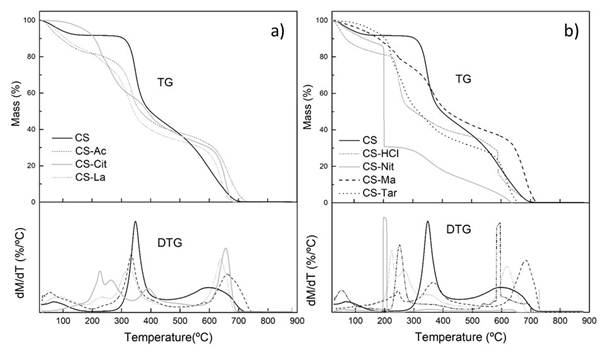
Fig.3. a),b). Thermogravimetric and differential thermogravimetric curves of chitosan and chitosan films
The TG curve of CS exhibited an endothermic effect (ΔH = 49,6 J/g) attributable to water release at approximately 25-183 ºC with 8,2 % weight loss. The second decomposition stage onsets at 183 ºC and extends to 457 ºC, with 49,5 % weight loss and maximum decomposition velocity (DTG curve) at 348 ºC. It is exothermic (ΔH = -174,8 J/g) and is attributed to a complex process including dehydration of saccharide rings, depolymerisation and decomposition of the acetylated and deacetylated units of the polymer. It has been shown that the energy associated with the latter is strongly dependent upon the degree of deacetylation of the polymer. The higher the degree of deacetylation of chitosan, the higher the amount of energy evolved. 33,34 The third decomposition stage is a thermo-oxidative process that starts at 497 ºC, with maximum velocity at 597 ºC and a weight loss of 42,3 %, leaving no residue behind.
The thermal degradation of the films showed considerable reduction in thermal stability when compared to chitosan powder. CS-Ac film presents a continuous weight loss between 25 and 199 ºC, peaking at 57 ºC with 11 % weight loss due to the evaporation of water and residual solvent. The second degradation stage is exothermic (ΔH = -219,8 J/g) with maximum decomposition velocity at 333 ºC and a weight loss of 50,7 %. The third degradation step is a thermo-oxidative process and appears at 497-735 ºC with 38,3 % weight loss. This is consistent with literature data, although the maximum decomposition temperature might vary between reports because of the recognized influence of the deacetylation degree and molecular weight of the chitosan used on its degradation parameters.28,34,35
From the DSC thermogram of CS-Ac film it was possible to determine the glass transition temperature (Tg) as Tg = 167 ºC (fig. 4). It is well known that there is a great dispersion in the chitosan Tg values reported in the literature. For instance, Sakurai et al.36 obtained a Tg value of 203 ºC using DSC, while other authors using thermomechanical analysis reported values ranging from −23 to 67 C for samples with different water content, indicating the plasticizing effect of water.37 Additionally, it must be taken into account that chitosan is a semicrystalline polysaccharide obtained from a natural polymer and its crystallinity, deacetylation degree and molecular weight influence Tg. In spite of this, it is worth mentioning that the Tg value obtained here is comparable to the Tg = 170,9 ºC reported by Martínez-Camacho et al.38
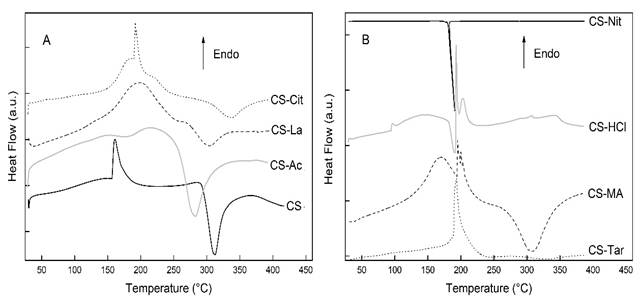
Fig. 4 a), b). Differential scanning calorimetry curves of chitosan and chitosan films prepared from different organic and inorganic acid solutions
The thermograms of the rest of the films prepared from organic acid solutions CS-Cit, CS-La, CS-Ma and CS-Tar exhibited a more complex pattern in which polymer degradation starts at lower temperature, indicating a decreased stability as compared with CS. The films prepared from inorganic acid solutions, CS-HCl and CS-Nit films presented the lowest thermal stability. The maximum degradation velocities of the stage at which polymer degradation occurs were located at 250 ºC for CS-HCl and 198 ºC for CS-Nit. In the latter, there is almost 70 % weight loss in this degradation stage, in which after a moderate rate at lower temperatures, the film exhibited and abrupt increase in degradation velocity with maximum at 198 ºC. The DSC thermogram shows the very narrow exothermic effect (ΔH = -219,8 J/g) associated to this process. This lower thermal stability of CS-Nit films is due to the exothermic oxidative reactions produced on the polysaccharide by the HNO3 molecules generated during the first step of the degradation process. This effect has been previously reported for the degradation of other organic nitrates.39
Conclusions
It has been shown that the nature of the acid used to prepare the chitosan solution influences the properties of the resulting chitosan film. The differences in the infrared spectra of films reflect differences in the intermolecular and intramolecular interactions with the acid. The amorphous character of films is evidenced in the X-ray diffractograms, which also differ between them. These characteristics are reflected in the thermal behaviour of films. They were all less thermally stable than the starting chitosan powder and showed different degradation patterns. Films prepared with organic acids were more thermally stable than those obtained with the strong inorganic acids, being the one prepared with nitric acid the less stable one. This is the result of oxidative reactions produced by the nitric acid released during the first degradation step.
The present study indicates that the selection of the acid solvent to prepare chitosan films is an important process variable with influence on theircrystallinity and thermal stability. The influence of the acid on other relevant properties of films, such as permeability to oxygen, antimicrobialcapacity, biodegradability and biocompatibility are at present under investigation