INTRODUCTION
As defined by the NASA Human Research Program, NASA’s highest-priority risks are provided on line at https://humanresearchroadmap.nasa.gov/. Separately, specific information and research priorities for deep-space missions are available in the National Academies of Sciences, Engineering, and Medicine. 2018 Mid-Term Report.1 More research is needed to understand the interrelationships between physiological adaptation to weightlessness including structural and functional changes of the musculoskeletal, cardiovascular, cerebro-ocular, immune systems as well as effects on cognitive performance, sleep, and group dynamics. Adding to the complexity of (mal) adaptations to long-duration missions is the large variability in individual responses with some crewmembers experiencing extensive symptoms while others are relatively unaffected. Genetic and/or psychological differences may be part of the underpinnings of these individual differences in resilience to extended space missions. Team performance and effectiveness of multinational crews are other factors and importantly, new and reliable means of assessing work performance in space is a significant priority.
For crew physical health and countermeasures, new smaller integrated exercise devices are required for deep-space missions to minimize size and increase efficacy. This is a high priority in order to optimize physical activity and develop a multisystem countermeasure that maintains cardiovascular health and musculoskeletal strength and prevents blood and fluid shifts towards the head. Determining the basic mechanism of adaptations and clinical significance of regional vascular/interstitial fluid pressures, due to altered Starling pressures as hydrostatic pressure gradients are abolished in space, continues to be important. Wearable lower-body negative pressure (LBNP) suits and LBNP exercise devices are possible mitigation strategies that should be included in this priority area. LBNP provides fluid shifts towards the feet but also displaces the subject into the device. Therefore, if combined with a solid base, ground reaction forces are generated under the feet.
Artificial gravity (AG) as a multisystem countermeasure seems the ideal countermeasure for deep-space missions. AG has, and is currently, is studied across the world in combination with bedrest trials as terrestrial analogues of weightlessness, including extended periods of time up 180 days. This form of AG is produced by centrifugation, which can be generated by the pedaling action of an incorporated exercise bike thus creating self-generated AG in combination with exercise.2
It is important for integrated countermeasures in space to reproduce normal musculoskeletal loads and blood/fluid shifts that occur on Earth and to which the body is adapted. Such integration of countermeasures may maintain not only the structure and function of muscle and bone, but also cardiovascular and cerebral health. Separately, hardware is needed to activate and maintain muscles that provide low-level but prolonged contractions common to upright posture on Earth. The addition of virtual environment (VE) technology is recommended for multisystem exercise hardware to improve the exercise experience for crew members and to foster adherence to exercise regimens. VE technology may also contribute to crew behavioral health and performance in small, confined habitats. Ground-based (e.g., bedrest) studies are an important initial step in developing an integrated, multisystem countermeasure combining exercise and foot-ward blood and fluid shifts.
TERRESTIAL ANALOGS
Efficacy of countermeasures can to tested in terrestrial analogue environments. True weightlessness is provided for 20-25 seconds during parabolic flight by letting an airplane perform a free fall.3) Long-term simulation of weightlessness is most commonly performed by 6 degrees head-down tilt bedrest to reproduce the blood/fluid shift towards the head and mechanical unloading of the body (at least in the axial 1Gz direction). Bedrest studies can be extended for several months.4 Other analog environments include water immersion in which the hydrostatic pressures of the surrounding water cancel out the hydrostatic pressures of the vasculature and rather acutely simulates effects of weightlessness.5 Dry immersion is used when simulation is required for shorter periods of time.
A common shortfall of many bedrest studies is the lack of inclusion of a proper control condition. In this regard, many bedrest trials have compared an intervention to passive bedrest and thus, find that any intervention or countermeasure is better than passive bedrest. As a minimum, any countermeasure employed in a bedrest trial should, as a minimum, be tested against current standard of care in space which is an ambitious exercise-program.
COSMIC RADIATION
One effect of spaceflight, which is difficult to simulate on Earth, is the increased and altered composition of background radiation. Space radiation risks to human’s highlights another major research topic that is ranked “highest priority,” a priority that includes a deeper understanding of deep-space radiation effects on biological and physical systems. Acute toxicities to radiation may be a risk for the central nervous system (CNS) when moving beyond low Earth orbit and the Van Allen Belt. Evidence suggests that there may be some acute CNS toxicity (such as cognitive dysfunction) in animals that have been exposed to high-energy particle radiation even at low doses;6) this work clearly warrants verification and additional study, particularly from a mechanistic perspective to assess whether similar effects might occur in humans. Additionally, a concern regarding radiation is elevated post-flight, life-time risk of cancer. Hence, the consensus for cosmic radiation research is that the key will likely be to limit exposure. Thus, faster transition time and adequate shielding of spacecraft and habitats at the site of destination (e.g. moon or Mars base) are needed.
GRAVITY OF EXERCISE FOR A MISSION TO MARS
In general terms, mechanisms responsible for physiologic adaptations to the microgravity of space include:
Loss of hydrostatic (gravitational) pressures within blood and fluid columns of the body such as arterial and venous blood (Fig. 1), cerebrospinal fluid and lymph.
Loss of body weight and greatly-reduced mechanical loads.
Decreased sensory inputs.
Altered Starling, transcapillary and lymphatic forces/transports.
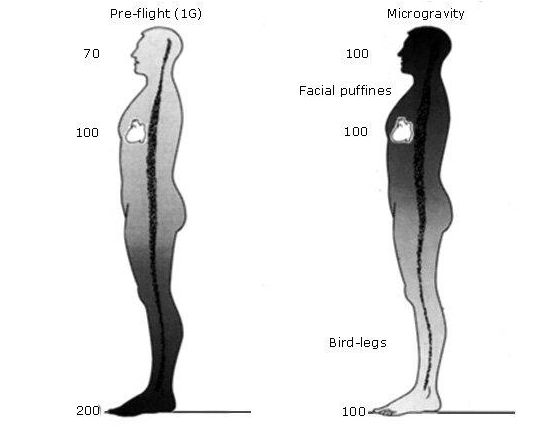
Fig. 1. Hypothetical arterial blood pressures (mm Hg) while upright in 1G and during microgravity. Modified from.7
Normal daily activity on Earth involves about 16 hours of upright posture with activity. This is commonly comprised of about 8-10 hours of seated posture, 1-4 hours of walking and perhaps 1 hour of strenuous exercise such as sports and fitness activities. The remaining part of the day consists of 8-9 hours of sleep without axial loading. In actual microgravity, external compression of body surface areas is minimal. The greater compression of tissues on Earth due to body and tissue weight increases interstitial fluid pressures and probably dehydrates these tissues with weight bearing due to greater interstitial flow into the microcirculation.7 The recently-defined Space Flight Associated Neuro-Ocular syndrome, or SANS, is evidenced by optical disc edema along with globe flattening, dilatation of the optic nerve sheath, choroid and retinal folds, deepening of the Bruch membrane opening, and ultimately refractive shift errors.8,9) SANS is predominantly seen in crewmembers during and following long-term (> 6 months) missions.10 Over the last 40 years, visual changes reported anecdotally in astronauts were thought transient with little operational impact. As mission durations have lengthened, reports of changes in astronauts’ visual function and ocular structure during spaceflight have increased and these changes are now defined as SANS.11
More recently, adding to the SANS and SANS-related findings are cerebral remodeling12 and potential cognitive consequences. SANS and SANS related findings may be maladaptive outcomes of microgravity, and similar to bone loss and muscle atrophy, these changes may persist long after return to Earth.13) Longer-term, follow-up studies will help determine if these changes are permanent.
Although the etiology of SANS remains unknown, microgravity-induced, headward blood and fluid shifts are considered the primary causative factor. Other contributors such as radiation, decreased overall cardiovascular health, elevated cabin CO2, high salt diet, and perhaps resistive exercise are all implicated as potential secondary contributing factors. Because the most important component of SANS is likely a headward fluid shift, a compelling countermeasure strategy is reversal of this blood and fluid shift.14) We have proposed many forms of LBNP to reproduce gravitational blood pressures and fluid shifts along with physiologic loading associated with upright posture on Earth. These concepts include a collapsible LBNP with treadmill, stair stepper or other exercise device. Other possible concepts include a mobile LBNP suit (Fig. 2), seated LBNP and self-generated LBNP (Fig. 3) to provide an integrated countermeasure. These concepts address the primary mechanisms of space-flight deconditioning due to loss of hydrostatic pressures within fluid columns of the body, cerebrospinal fluid and lymph, loss of body weight and greatly-reduced mechanical loads, decreased sensory inputs, and altered Starling or transcapillary pressures. Previous research documents that LBNP is effective to protect many other physiologic systems that are affected by microgravity.
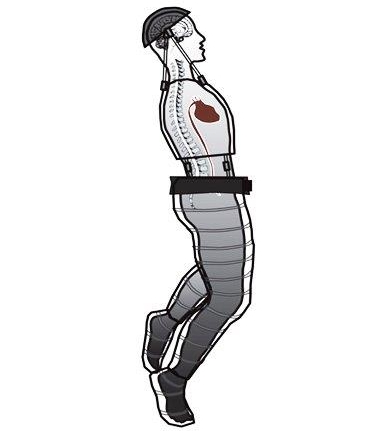
Fig. 2. Schematic of wearable LBNP-device. LBNP is generated from a portable vacuum powered by a battery. In addition to generating blood and fluid shifts towards the feet, mechanical forces are also generated by the vacuum. The negative-pressure trousers are attached to a vest and a cap to distribute the mechanical loads to the entire axial system. Use does not interfere with daily activity in space. Duration is limited by battery lifetime. (Petersen et al unpublished, with permission).
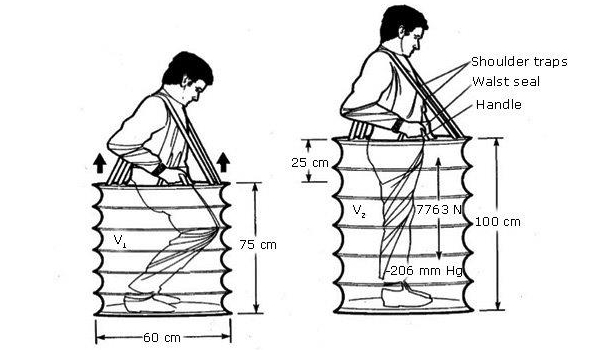
Fig. 3. Self-generated LBNP exercise device in microgravity. LBNP and force calculations are theoretical, based on expansion of a 60 cm diameter, air-tight cylinder from 75-100 cm in length. In practice, the device exhibits some leakage of air and some radial collapse of the cylinder occurs at high levels of effort (highly negative LBNP levels). Thus, few if any subjects can exert over 5000 N of footward force. Reproduced from.15
Conclusions and recommendations for future work
Effective countermeasures to maintain the health and well-being of crew members during prolonged spaceflight are best developed using an integrated physiologic approach. Future spacecraft designs for deep space are constrained by limited volume, food, water, shelter and other resources. Thus, it’s important to understand the highest risks and to direct research into these areas. Because effective hardware for artificial gravity by centrifugation may be decades away, we propose use of exercise within LBNP as means to simulate multiple beneficial effects of gravitational stress including blood/fluid shifts towards the feet and mechanical loading of the body. LBNP-devices can be configured as wearable devices to reduce mass or combined with exercise devices to increase efficacy of exercise in weightlessness.